Open Access
REVIEW
Nigerian Biomass for Bioenergy Applications: A Review on the Potential and Challenges
1
Department of Mechanical Engineering, Nile University of Nigeria, Abuja, 900001, Nigeria
2
Department of Petroleum and Gas Engineering, Nile University of Nigeria, Abuja, 900001, Nigeria
3
Department of Mechanical Engineering, Landmark University, Omu-Aran, 251103, Nigeria
4
Department of Industrial Chemistry, University of Ilorin, Ilorin, 240003, Nigeria
* Corresponding Author: Adekunle A. Adeleke. Email:
(This article belongs to the Special Issue: Advanced Renewable Mineral and Energy Materials)
Journal of Renewable Materials 2023, 11(12), 4123-4141. https://doi.org/10.32604/jrm.2023.043915
Received 15 July 2023; Accepted 13 September 2023; Issue published 10 November 2023
Abstract
Nigeria, often referred to as “the giant of Africa,” boasts a sizable population, a thriving economy, and abundant energy resources. Nevertheless, Nigeria has yet to fully harness its renewable energy potential, despite its enormous capacity in this field. The goal of this review paper is to thoroughly examine the difficulties and untapped opportunities in utilizing biomass for bioenergy production in Nigeria. Notably, Nigeria generates substantial volumes of biomass annually, primarily in the form of agricultural waste, which is often either discarded or burned inefficiently, resulting in significant ecological and environmental damage. Therefore, an efficient approach to reducing pollution and transforming waste into wealth involves converting these biomass resources into energy. This work critically examines the status of biomass utilization for energy applications in Nigeria and highlights the bottlenecks that impede its widespread adoption. The review emphasizes the economic and ecological advantages of biomass utilization over traditional waste treatment methods. Additionally, it underscores the appeal of biomass as an industrial fuel source, particularly considering the current high cost of fossil fuels in contemporary Nigeria. Relevant literature on biomass, energy, agricultural waste, fossil fuel, and calorific value in the context of Nigeria was reviewed by utilizing a thorough search technique in key scientific databases. The analysis did not include any non-English publications. The findings of this research provide valuable insights into the challenges faced in maximizing Nigeria’s biomass potential and offer strategic recommendations to promote the use of biomass for bioenergy development. This review paper will assist a wide range of local and international readers, as well as industries interested in green and bioenergy, in making informed decisions regarding the most suitable types of biomass for biofuel production.Graphic Abstract
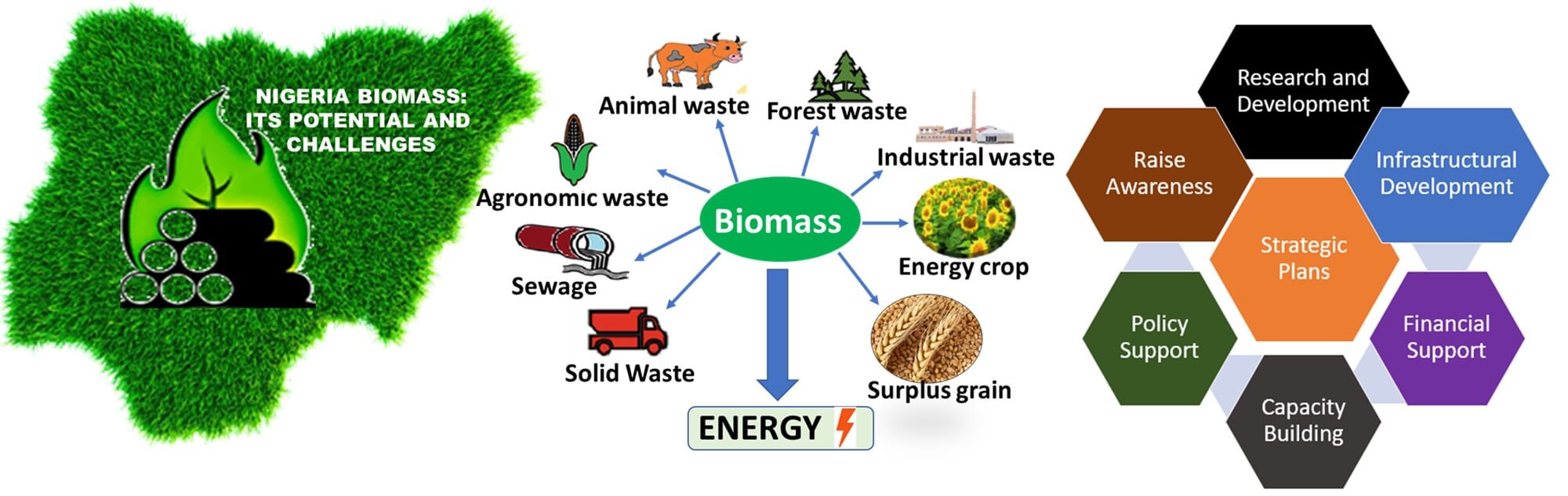
Keywords
Nigeria, the giant of Africa, is predicted to have a population of 352.67 million by 2030, with a growth rate of up to 4%. In 2022, its estimated population was 220 million, with a GDP of 440.8 billion US dollars. Nigeria is recognized as the most populous country and the biggest economy in Africa [1]. As the largest contributor, accounting for 90% of foreign exchange, the country’s energy sector, especially the crude oil subsector, has made a significant contribution to the expansion of the economy [1–3]. The natural resources of Nigeria are plentiful. However, despite its wealth of both renewable and non-renewable energy resources, Nigeria has yet to fully realize its potential in the renewable energy industry [4,5]. Renewable energy sources in the country include solar radiation with an insolation range of 3.5 to 7 kWh/m2/day, wind with a top speed of 4 m/s at a height of 10 m, and biomass from a variety of sources shown in Fig. 1 [1,6].
Figure 1: Major sources of biomass [6]. Reprinted with Springer’s approval (Order code: 501830398)
Biologically derived complex renewable material with significant chemical variability is referred to as biomass. It is divided into five groups: biomass from wood, biomass from plants, biomass from water, biomass from animal and human waste, and biomass from mixtures [1,7]. According to the Food and Agriculture Organization (FAO) in 2023, Nigeria’s land area spans 92.377 million hectares, of which 70.8 million hectares are utilized for agricultural activities. Nigeria generates millions of tons of various types of biomasses annually, mostly in the form of agro-industrial wastes as shown in Fig. 2, which are either left to litter the environment or burnt ineffectively, leading to serious environmental pollution [8,9]. To curtail this crucial issue, converting these biomasses into energy can serve as an effective solution to reduce environmental pollution. Adopting this ‘from waste to wealth’ (energy) approach has greater benefits from the economic and ecological standpoint as opposed to waste treatment, which incur financial costs. Moreover, given the high price of fossil fuel in Nigeria, utilizing biomass as fuel could potentially be an attractive option for industries. Regrettably, the direction of biomass development for energy applications in Nigeria has not been thoroughly explored [10]. Thus, this review article generously investigates challenges bottling potential of Nigeria biomass. This work also offers strategic advice to promote biomass use in Nigeria for advancement of bioenergy. This review will help local readers on how to harness some hidden Nigeria biomass potential. Also, this paper will assist broad local and international readership and industries with an interest in green/bioenergy in making decision on the best type of biomass for making biofuel.
Figure 2: Categories of various agro-industrial wastes. Reprinted with permission from [9] copyright (2022) MDPI
This review study employed the main scientific databases as shown in Fig. 3 to thoroughly search for suitable literature using keywords biomass, energy, agricultural wastes, fossil fuel, and calorific value in single or in combo with the word Nigeria. Publications in any language other than English language were removed from the depository of literature.
Figure 3: Review methodology scheme. Adapted from [11,12] with Elsevier’s approval (Order code: 5590210021832)
Agba et al. [13] estimated that Nigeria has bio-energy reserves/potential reaching 1.2 Peta Joules (PJ). According to Simonyan et al. [8], Nigeria possesses the biomass resources enough to produce up to 47.97 million metric tons of oil equivalent (Mtoe) annually. In contrast, Olanrewaju’s team [14] in their study reported a residue energy potential estimate of 49 Mtoe which was 1.79 Mtoe greater than that of Simonyan et al. [8]. It was further accentuated that the country can generate up to 1.2 million metric tons of biomass per day, according to Mshelia [15]. Although Nigeria has a huge potential for biomass-based bioenergy generation, its current output is still rather small [16]. Ezealigo’s research group [16] explored the prospect of biomass for electricity and biofuel production in Nigeria. Their findings highlighted that biomass would contribute significantly to the country’s energy mix. The study also suggested that non-food options, such as agricultural residues, are more sustainable for bioenergy generation. Unlike conventional food crops, these residues are cheaper, abundant, and do not compete with marginal or forest land, preserving biodiversity. The authors also pointed out several challenges that must be addressed to fully realize this potential. Of key concern in the present study were the issues of poor information on feedstock and inadequate research and development. The importance of having comprehensive information on potential feedstock for the development of biofuel plants was emphasized. A certified database for each potential feedstock is needed, which should be based on detailed characterization including proximate, compositional, and thermal analysis as this information would be required to identify feedstock suitability and potentials for other applications, as well as to modify crops to yield more oil. Having this information as well can guide investment decisions and avoid complexity in the selection of feedstock. The present research investigated feedstock suitability and detailed characterization. They suggested an integrated strategy that calls for the establishment of the Nigerian Biofuel Corporation through a Public-Private Partnership and the creation of the Nigerian Network on Bioenergy to develop a sustainable biofuel business in Nigeria. Sensitization is as well necessary across all stakeholders, from the government to farmers, investors, research, financial institutions, and end users [16].
4 Potential and Energy Value of Nigerian Biomass
Biomass-derived fuels can be classified into different generations based on the feedstock used in their production [17]. These generations include first, second, third, and fourth generation fuels. The majority of biorefineries emerging in Nigeria currently rely on first generation biomass feedstocks, which are primarily derived from food crops. Fortunately, Nigeria has a diverse range of crops that could be utilized as potential feedstocks for biofuel production. Sokan-Adeaga et al. [2] mentioned cassava, sugarcane, rice, sweet sorghum, palm oil, groundnut, and palm kernel as some of the potential crops. Notably, around 95% of biofuels used globally are made from crops that can be eaten [18]. This has raised concerns about the food vs. fuel dilemma, which underscores the need for sustainable production of crucial crops to address this issue [19].
An alternative is the use of second-generation feedstocks, which are typically derived from lignocellulosic biomass. These feedstocks can be obtained from various sectors, such as agriculture, forestry, and industry [20]. Using lignocellulosic biomass has the advantage of requiring less land use and having a lower impact on the food sector [21]. According to Akogun et al. [10], agricultural waste in Nigeria is a promising resource that can potentially cater to both environmental concerns and energy requirements. However, in Nigeria, the potential of using crop residues as feedstock for biofuels has not been fully exploited, limiting the realization of their energy potential [16]. Jekayinfa et al. [1] investigated several promising biomass resources that could be used to generate energy in Nigeria. These included residues from various crops such as maize, cassava, cowpea, cocoa, coconut, coffee, groundnut, millet, oil palm, plantain, potato, rice, sorghum, soybean, sugarcane, wheat, and yam. Table 1 displays the chemical make-up of these agricultural wastes in Nigeria as stated in their study [1]. These agricultural waste products are classified as herbaceous biomass, a class of biomass. Tursi [22] identified four other biomass groupings that are categorized according to ecological or vegetation types: woody biomass, aquatic biomass, animal and human waste biomass, and biomass combinations.
Another significant source of biomass resources is the forests in Nigeria and this can greatly contribute to the country’s energy needs. As reported by Simonyan et al. [8], about 11.3 percent of Nigeria’s total land area (about 10 million hectares) is made up of forest reserves and the distribution of this forest plantation across the nation is shown in Fig. 4. This estimate is corroborated by studies by Ben-Iwo et al. [17] and Sokan-Adeaga et al. [2], which show that Nigeria has about 1,160 forest reserves that encompass 10,752,702 hectares, or about 10% of the country’s land area. Nigeria’s forest residue is thought to have an energy potential of about 8.68 Mtoe (363 PJ), which can be derived from roughly 19 million tonnes of forest leftovers, according to Olanrewaju et al. [14]. The figure made by Simonyan et al. [2], which was based on production data from 2010 and only amounted to 0.02 GJ, is greater than this one.
Figure 4: Spread of Nigeria’s plantations of forests, where A = Ondo, Lagos, Oyo, Osun, Ogun, and Ekiti state; B = Anambra, Abia, Ebonyi, Imo, Edo, Enugu and Delta state; C = Benue, Rivers, Cross Rivers, Bayelsa and Akwa-Ibom state; D = Nasarawa, Kogi, Kano, Niger, Taraba, Kebbi, Kwara, Kaduna, Adamawa and Plateau state. Reprinted with permission from [25] with Elsevier’s approval (Order code: 5625830947902)
According to Ezugwu research team [23], the lumber industry is associated with considerable biomass resources, particularly wood wastes and sawdust. There is also significant potential for fast-growing aquatic biomass due to the country’s large coastal regions spanning over eight states [16]. Commonly found in Nigeria are three fast-growing aquatic weeds: water lettuce, water hyacinth, and brackenfern [1,16]. Given their high cellulose and hemicellulose content and low lignin content, these aquatic weeds are effective as biofuel crops, according to Kaur et al. [24]. They also have very rapid reproduction rates. When contrasted with land crops, aquatic biomass typically has the benefit of providing more resources per hectare [1].
The efficient and rational use of biomass as fuel requires the characterization of its parameters such as MC, AC, VM, and FC, among others [26]. Umar et al. [27] also supported this notion, stating that it would be ideal to carry out characterization tests to see whether biomass feedstock may be used as fuel for energy production. Lin’s team [28] highlighted the need to understand the effects of biomass properties on the composition and yield of thermal products for optimizing both biomass and conditions for thermal conversion. A thorough comprehension of the physicochemical characteristics of lignocellulosic biomass and the methods used to analyze these properties is crucial for the effective design of thermochemical or biochemical processing methods for biomass [20]. Banerjee et al. [29] further highlighted that inadequate determination of the physicochemical properties of lignocellulosic biomass samples can result in low quality and quantity of pyrolysis yields. Notably, numerous characterization studies have been conducted to evaluate various biomass resources, including investigations into their elemental properties and energy contents [30]. For instance, Mythili et al. [31] explored the suitability of 25 lignocellulose materials for bio-oil production. Chang [32] analyzed the possibilities of employing oil palm’s empty fruit bunch (EFB) as a feedstock for the manufacturing of bio-oil and contrasted the characteristics of the bio-oil with that of fuel. Using common characterization techniques like proximate and ultimate analysis, metals analysis, and the ash fusion test, Akinrinola et al. [5] investigated the combustion behavior of five potential energy crops and woods from Nigeria, including Terminalia superba, Gmelina arborea, Lophira alata, Nauclea diderrichii, and palm kernel expellers (PKE), and then contrasted their findings to some U.K. biomass. Eze-Ilochi et al. [33] investigated the characteristics of 10 indigenous biomass wastes from Nigeria and compared their metal, sulfur, proximate analysis, calorific value, and morphological compositions to coal. Bambara nuts, bush mango nuts, cassava stalks, cocoyam peels, detar shells, empty fruit bunches, maize stalks, mango seed shells, sugarcane bagasse, and wild cassava peels are among the biomasses. Singh et al. [34] profiled five lignocellulosic biomass samples, namely Impereta cylindrica, Eragrostis airoides, Typha angustifolia L., Arundinella khasiana Nees ex Steud, and Echinochloa stagnina (Retz.) P.Beauv, using proximate, ultimate, and compositional analysis to fathom their compositional and structural profile.
Balogun’s team [30] examined the combustion properties and elemental composition of two biomass resources, namely araba (Ceibo pentandra) and sorghum husks. Padilla research team [35] used Fourier Transform Infrared Spectroscopy (FTIR) and thermogravimetric analysis (TGA) to explore the manufacture of charcoal utilizing maize cobs as a feedstock. Another group [36] investigated the physicochemical characteristics and compositions of Swietenia macrophylla (mahogany) wood, and the amount of bio-oil obtained was quantified to determine how useful it could be for bioenergy production and other industrial uses. Wang et al. [37] considered the potential of using seaweeds as a feedstock for biofuel production through the process of pyrolysis. Umar et al. [27] evaluated the suitability of oil palm trunks and oil palm fronds as feedstock in thermochemical processes using ultimate analysis, proximate analysis, calorific value, thermogravimetric analysis (TGA), field emission scanning electron microscopy (FESEM), and X-Ray fluorescence (XRF). Awoyale et al. [38] investigated the impact of three pretreatment methods on five Nigerian lignocellulosic biomass sources. The biomasses studied were corn cobs, cassava peels, sugarcane bagasse, rice husks, and white yam peels. Proximate and ultimate analysis, FTIR, BET, XRD, and SEM were employed to characterize both the raw and pretreated biomass and evaluate the impact of the pretreatments on the biomass. Efetobor’s team [39] examined the properties of elephant grass as a biomass source for bio-oil production. Olawale et al. [40] investigated the potential of plantain leaves, stem and flowers for bio-oil production. Ahmmed et al. [41] explored the use potato (solanum tuberosum) peel waste as a feedstock for bio-oil through pyrolysis.
A corn cob is a byproduct of agriculture made from maize and is still attached to the ear where the kernels develop [42]. Over 1.2 billion people in sub-Saharan Africa and Latin America depend on corn, scientifically known as Zea mays, as their primary source of food [43]. Maize is the most produced grain globally, with Nigeria ranking the 13th producer of corn worldwide and leading in Africa. The current global production of corn is estimated to be 1,151 million tons, with Nigeria contributing approximately 12 million tons, as reported by the International Production Assessment Division in 2023. Corn is grown throughout the country, with Kaduna being the main production base. However, its waste, including corn cobs and stovers, are often burnt or left to litter the streets, which is not environmentally friendly. By logical inference, one can say that as long as corn production continues in Nigeria, there will be a sustained generation of corn cobs. Thus, these residues can be used to generate energy and develop new materials The energy value (heating value) and proximate and ultimate analyses of maize cob for various applications have been published in earlier studies. Table 2 displays the maize cob’s proximate, ultimate, and calorific values from earlier studies. The suitability of corn cob as a biomass feedstock for slow pyrolysis was examined by Shariff et al. [44]. This was accomplished utilizing heating value, pH, lignocellulosic determination, proximate, elemental, and thermogravimetric (TG) analyses. According to the proximate analysis, the feedstock made from maize cobs had a high VM of 87.76%, a low ash level of 1.05%, a 7.14% MC, and 11.09% FC. The results of the elemental analysis revealed that the feedstock made up of maize cobs had 43.81% carbon, 6.54% hydrogen, 0.77% nitrogen, 0.69% sulfur, and 48.19% oxygen. Corn cob feedstock, when utilized as a feedstock for the pyrolysis process, is environmentally beneficial due to its low nitrogen and sulfur percentages. The lignocellulosic examination of maize cob feedstock showed that it had a cellulose content of 45.88%, hemicelluloses of 39.40%, and lignin of 11.32%. Corn cob feedstock SEM examination revealed a tight structure without any pores.
Anukam’s research group [42] studied the fundamental properties of maize cob to assess the gasification potential. Their results were examined in relation to gasification using information that had previously been published. Its three primary elemental components (C, H, and O) as well as the weight percentages of its three basic features, including moisture, volatile matter, and fixed carbon levels, were calculated. By using FTIR to identify the atoms in the structure of corn cobs, it was discovered that the main functional groups of these atoms were -OH, C-O, C-H, and C=C. A maize cob entirely thermally decomposes at heating rates of 20 °C/min and temperatures about 1000°C, according to TGA. The evaluation of CC in terms of its gasification-related characteristics was done after a thorough examination based on fuel analysis. Their research showed that CC is an appropriate biomass fuel for gasification.
A study by Liu’s team [45] analyzed and differentiated the thermal and physicochemical properties of corncob-derived biochars from those of sawdust and cornstalk. According to their investigation, the corncobs had a moisture content of 11.7%, an ash content of 2.9%, a volatile matter content of 69.5%, and a fixed carbon content of 15.9%. In addition, they recorded the corncobs’ relative concentrations of carbon, hydrogen, nitrogen, and oxygen, which were 48.12%, 6.48%, 1.89%, and 43.51%. Additionally, it was said that the H/C and O/C atomic ratios were 1.43 and 0.64, respectively. The percentages of lignin, cellulose, and hemicellulose as well as the pH of the corncobs were all determined as part of the study.
The wood and furniture industries produce sawdust as a byproduct, and heaps of it are frequently burned off at disposal sites. Boluwaji et al. [48] reported that the process of transforming logs into lumber at sawmills leads to a significant amount of wood waste in the form of sawdust [48], found that the conversion efficiency typically ranges from 45%–50%, which means that around 55% of the wood material is left as sawdust. This, in turn, contributes to the accumulation of large piles of sawdust that are commonly observed around sawmills in Nigeria. There are numerous sawmills all around the country making sawdust readily available [43]. Sawdust can be effectively disposed of sustainably by converting it into energy in the form of bio-oil through fast pyrolysis [48].
Adegoke et al. [49] looked into the feasibility of pyrolyzing Gmelina arborea (sawdust) biomass in a fixed bed pilot-scale reactor to produce bio-oil. The bio-oil’s physical, chemical, and final analyses were carried out by accepted techniques, and the results showed that it had desirable qualities. Similarly, Boluwaji et al. [48] conducted a study to investigate the potential for bio-oil production from sawdust in Southwest Nigeria. These results from the two investigations indicate that sawdust is a promising resource for the manufacture of bio-oil and can lessen the environmental damage caused by conventional fuels. Mensah et al. [50] characterized Celtis mildbraedii sawdust as a potential feedstock for syngas production. The report of the proximate, ultimate, and higher heating values is presented in Table 3. The carbon content of sawdust ranges from 77.51% to 93.59% with its ash content as low as 0.08% [48].
Groundnut shells are the residue left over once the groundnut seed has been separated from its pod. Nigeria holds a prominent position in the global production of groundnuts, ranking as the largest producer of groundnuts in Sub-Saharan Africa and the third largest producer worldwide [52–54]. Groundnut, scientifically known as arachis hypogaea, is a leguminous oilseed crop grown in regions characterized by semi-arid and subtropical climates around the world. In Nigeria, groundnuts are primarily cultivated in the dry regions of the country, with major plantations located in Kaduna, Kano, Bauchi, Sokoto, Kwara, and Zamfara [55]. Nigeria, the largest groundnut-producing nation in West Africa, contributes 10% to global output, 51% of the regional output, and 39% of African output. According to Jekayinfa et al. [1], groundnut cultivation in Nigeria spans over 2.8 million hectares and yields roughly 2.4 million metric tonnes of the crop. However, groundnut waste constitutes a significant environmental concern in Nigeria, with some waste products used for animal feed, disposed of in landfills, or burnt [56,57] revealed that shells account for 27% of the groundnut waste generated. Duc’s team [58] opined that the presence of multiple functional components in groundnut shells makes them suitable for various applications. In this regard, turning groundnut trash into biofuel presents a more viable economic and environmental alternative.
Several researchers have explored the potential of groundnut shells for various uses. For instance, groundnut shell to biofuel conversion, particularly biodiesel and bioethanol, has been studied by [58]. Nyachaka et al. [59] investigated the production of bioethanol fuel from groundnut shell waste and evaluated its performance. Raphael et al. [60] studied the physicochemical and combustion properties of briquettes obtained from pyrolyzed biochar of groundnut shells. Varma et al. [61] explored the thermal degradation behavior and investigated the kinetic and thermodynamic parameters for the pyrolysis of peanut shells. The proximate, ultimate, and higher heating values of these studies are presented in Table 4.
Lemongrass, scientifically known as cymbopogon citratus, is a type of tropical perennial plant that is widely recognized for its fragrant oil. Its name is attributed to the distinct scent of the essential oil found in its shoots, which resembles that of lemons. Lemongrass is commonly grown in poor, marginal, and waste lands, as well as along bunds to serve as the live mulch. Additionally, the well-branched root system of the plant helps in conserving soil and water. Lemongrass has the potential as a high-biomass crop for producing biofuel [65]. The high-value oil content of lemongrass reduces the production cost of its biomass for biofuel. This suggests that lemongrass could be a valuable specialty crop for biofuel production [66]. Madhu et al. [67] conducted a study on the production of bio-oil from lemon grass through pyrolysis. The structural and elemental composition of lemon grass were determined. The VM, FC, MC and AC were reported to be 59.99%, 24.78%, 8.02%, and 7.21%, respectively. The ultimate analysis revealed a composition of 39.34% carbon, 5.81% hydrogen, 1.54% nitrogen, 53.30% oxygen, and 0.01% sulphur [67]. Investigations were conducted into the primary traits of the bio-oil generated and its production rate. The study’s findings indicated that bio-oil is a contender for biofuel even if its efficiency during engine combustion will be somewhat poorer due to the presence of moisture content and oxygenated constituent.
Cashew trees, which are members of the Anacardiaceae family and the genus Anacardium, are a versatile crop with significant economic value for many nations, including Nigeria. It is an evergreen, drought-resistant tree that is raised for its wood, apples, and nuts. About 27 states in Nigeria now have cashew farming, and output has greatly expanded in recent years. Enugu, Abia, Imo, Anambra, Ebonyi, and Cross River States are among the states in Nigeria that grow cashews. Other states that grow cashews include Oyo, Osun, Ondo, Ekiti, and Ogun States in the Southwest, Kwara, Kogi, Plateau, Benue, and Abuja in the North central, Nassarawa, Taraba, Niger, Kaduna, and Sokoto and Kebbi States in the North-western region of the nation. Bhavsar et al. [68] conducted proximate analysis of selected non-woody biomasses, including cashew leaves. The analysis revealed that cashew leaves had a volatile matter content of 65.33%, ash content of 6.9%, moisture content of 10.86% and 16.87% of fixed carbon.
5 Development of Biomass Technologies in Nigeria
Different types of fuel are made from biomass depending on the kind of feedstock, process of production and end use. Biomass technologies in Nigeria are at varying stages of development, with the majority currently in the research and development phase, and only a limited number advancing to the pilot and commercial stages [17].
Because wood has a high calorific content and little ash, it has been used as a fuel source for hundreds of thousands of years and continues to play a significant role in the world’s use of clean energy sources [69,70]. As a form of biomass energy, wood fuel offers several advantages. Unlike fossil which takes millions of years to form, wood can be continually replenished through sustainable forestry practices. When burned as fuel, carbon dioxide (CO2) that trees take throughout their growth is released back into the atmosphere. However, the carbon emitted during wood combustion is equal to the amount absorbed during the tree’s lifetime, resulting in a closed carbon cycle and making wood fuel carbon neutral. Wood is widely available across the globe, making it easily accessible for energy production. This accessibility contributes to energy security and offers opportunities for rural communities to harness their local resources for heating, cooking, and electricity generation. Wood fuel is the main household energy source in underdeveloped nations like Nigeria, where it provides about 37.4% of the country’s total energy consumption, making it the largest non-commercial energy source [2,71]. Over 76% of people in Nigeria use wood fuel for cooking, including charcoal and firewood. However, only 5%–15% of wood fuel can be converted into usable energy. It is critical to investigate the possibilities of switching to a more efficient fuel source given the widespread usage of wood fuel in Nigeria and its low performance. In addition, the rising demand for fuel wood creates a serious environmental risk by causing erosion and deforestation [72,73]. In Nigeria, it is crucial to harness and make accessible additional energy sources, while also taking into account biomass alternatives to replace wood as a residential energy source [74–76].
Gasification is the partial oxidation of biomass at high temperatures, often between 800°C and 900°C, to generate syngas, an amalgamation of combustible gas. The mixture consists of CO(g), H2(g), CO2(g), CH4(g), with traces of other hydrocarbons, H2O(g), and N2(g) from the air used in the reaction [8]. The low-calorific gas that is produced can be burned directly or utilized to feed gas engines and turbines to produce energy. It serves as a valuable feedstock for the manufacture of chemicals. Nigeria’s enormous biomass resources allow for the implementation of biomass gasification to produce biogas throughout the entire nation.
A 25 MW electric capacity rice husk gasification facility has been developed in Ebonyi state, in the southeast of Nigeria, because of cooperation between the state government and a UN organization. Several facilities, including streetlights, a primary healthcare facility, an information and communication center, and an existing palm kernel processing plant, receive their electricity from this plant. Another gasification facility has also been built in the same state to provide energy to nearby small-scale rice millers, the Ebonyi Agro-rice Mill, neighborhood merchants, and the Oferekpe mega water project [77,78].
Bioethanol is primarily produced through the fermentation of biomass, involving enzymatic breakdown by microorganisms at low pressure and temperature [79–81]. Series of studies have been done on bioethanol [82–84], with various feed stocks and current technologies [85–87]. Recent studies have given insights into the future prospects of bioethanol production [88–90]. Nigeria is making significant investments in the development of its bioethanol industry. Existing automobile biofuel projects can produce 1.8 million tons of feedstock and 59 MW of electricity annually in Agasha, Guma, Bukaru, and Kupto in the states of Benue, Gombe, and Benue respectively. Additionally, Ebenebe, Anambra State’s vehicle biofuel project, has a bigger capacity with 3.5 million tons of feedstock [91,92]. Nigeria is making significant investments in the development of its bioethanol industry. Existing automobile biofuel projects can produce 1.8 million tons of feedstock and 59 MW of electricity annually in Agasha, Guma, Bukaru, and Kupto in the states of Benue, Gombe, and Benue respectively. Additionally, Ebenebe, Anambra State’s vehicle biofuel project, has a bigger capacity with 3.5 million tons of feedstock [17,93,94]. The main feedstocks used in Nigerian bioethanol projects include cassava, sugarcane, and sorghum. However, it is imperative to avoid causing food crises while providing energy security because these feedstocks are key food crops. Nigeria has enormous uncultivated areas that might be used for energy crops, thus methods for boosting capacity are needed to keep bioethanol production going.
6 Main Challenges of Nigerian Biomass for Energy
Despite having a significant potential for its generation from numerous diverse sources available for biomass production, Nigeria’s population growth and economic development are increasing the demand for renewable energy. Unfortunately, the infrastructures for supplying and extending this essential energy, especially to rural areas, inadequate policy design and implementation, an inadequate regulatory framework, an unfavorable climate for investment, and inadequate access to technology, among other things, have been some of the major challenges encountered recently [95–97]. It is also clear that agricultural feedstock plays a significant role in Nigeria’s biofuel production process. However, due to pressure on valued trees for their economic and ecological relevance, forest resources including sawdust, wood chips, and bark are no longer available to produce biomass transformed into energy. According to a report, animal waste accounts for 2.17 EJ of the overall 5.81 EJ of the theoretical potential obtained from residue-based biomass, which is calculated as the sum of the energy potentials of agricultural residues and animal waste. The theoretical potential, which mostly depends on the availability factor of each biomass resource, which ranges from 0 to 1, is just a small portion of the technical potential that may be tapped for energy usage. The availability factor is applied because only a portion of the theoretically possible annual biomass potential could be harvested. According to reports, a range of values were employed due to the high degree of uncertainty surrounding the availability factor, including 0.10 and 0.30 for cereals, up to 0.40 for rice, and between 0.08 and 0.50 for sugarcane. The range was 0.10 to 0.25 for grass while it was 0.50 to 0.75 for wood residues. Assuming a generalized availability factor as one of the many difficulties impacting the use of biomass for energy in Nigeria, given the provided range of values’ poor quality [1]. Problems with Nigeria’s land tenure system have been cited as another important challenge. State ownership of land is established by the Land Use Act of 1978, which deals with the issue of the structure of land ownership in Nigeria. They held the land in their states in trust for the residents of that state since bioenergy facilities would need a large amount of land for the production of energy crops. If the approval of the state governors is not sought, this could continue to be a barrier to large-scale farming and prevent private investors and the federal government from having access to raw materials for the production of bioenergy [7,25,98]. The requirement for large upfront capital and continuous maintenance costs, as well as widespread hostility to using biogas as an energy source, particularly in rural areas, are further characteristic limiting factors. Because it is a novel technology and has a higher value than the traditional fossil fuel generating methods, it may demand a large initial investment [98]. The high cost of the enzymes required to make biogas from cassava, the stress placed on food crops cultivated on arable lands for the production of biogas, and a dearth of clarity surrounding the utilization of energy crops like jatropha as raw materials for large-scale biofuel production are a few additional significant problems that hinder the use of biofuel resources in Nigeria [19].
7 Strategic and Feasible Recommendations to Simulate the Local Conversion of Biomass into Energy
By and large, as seen in the previous sections, biomass has remarkable potential along with numerous benefits like reducing greenhouse gas emissions and energy insecurity. However, sequel to the challenges accentuated in the foregoing section, this segment aims to provide strategic recommendations on how to encourage the use of biomass in Nigeria, and overcome its challenges, fostering a transition to a more sustainable energy landscape. The recommendations shown in Fig. 5 can pave the way for a greener and more sustainable future for Nigeria [16,25,99]. The recommendations are discussed in the following subsections.
Figure 5: Recommended action for a greener and more sustainable future for Nigeria
To inform the public, decision-makers, and corporations about the advantages of biomass as a renewable energy source, launch focused awareness campaigns. Draw attention to its potential for lowering deforestation, enhancing air quality, and offering rural people decentralized energy alternatives [16].
Encourage the use of biomass for energy production by implementing supportive policies and regulatory frameworks. This could include grants for biomass-based initiatives, tax incentives, and feed-in tariffs. To obtain the required permits and licenses, establish precise guidelines and simplified processes [25,99].
Spend money on projects that conduct research and development to improve biomass conversion technologies like gasification, pyrolysis, and anaerobic digestion. Encourage collaborations between academic institutions, research facilities, and the private sector to promote innovation and create biomass energy solutions that are affordable [16,25].
Develop the skills of local communities, technicians, and business owners in biomass energy systems by offering training programs and workshops. Concentrate on knowledge transfer, upkeep, and biomass plant operation to ensure long-term sustainability and employment development [25,99].
Establish financing programs for biomass energy projects, such as low-interest loans or venture capital funds. Encourage collaborations with financial institutions to help the biomass industry expand and provide potential investors and project developers with access to cash [16,25,99].
7.6 Infrastructure Development
Invest in the distribution, processing, and production infrastructure required for biomass. Establish specialized biomass supply chains with collecting, storage, and transportation infrastructure. This will guarantee that biomass resources are used effectively and open doors for farmers and rural communities [16,25,99].
7.7 Public-Private Partnerships
To encourage investment and innovation in the biomass energy sector, and foster cooperation between the public and private sectors. Encourage collaborations between businesses in the energy, agriculture, and technological sectors to build integrated value chains and guarantee market viability [99].
Participate in neighborhood groups in biomass energy initiatives to promote ownership, societal acceptance, and long-term viability. Encourage community involvement in decision-making, provide just pay for land usage, and make sure that benefits are distributed fairly [99].
Launch demonstration facilities and pilot programs to demonstrate the viability of biomass energy options. To inspire confidence in prospective investors and stakeholders, highlight accomplishments and discuss lessons learned [25,99].
7.10 International Collaboration
Participate in international partnerships and collaborations to take advantage of technical know-how, information sharing, and funding. To benefit from their expertise and tailor best practices to the Nigerian situation, establish collaborations with nations or organizations that have successfully established biomass-based energy systems [16,25].
The prospects and challenges of producing bioenergy from biomass have been reviewed. Various biomass such as corncob, lemon grass, wood dust and others have been identified as prominent Nigeria second generation feedstock for bioenergy. Biomass technology varies in stages of development though the majority are currently at the research and development stage. Only a few are in the advanced pilot and commercialization stage. Gasification, bioethanol production and wood fuel for domestic energy are currently on a rampage in Nigeria. The major challenges are little or no infrastructure for biomass energy production, inadequate policy design and implementation, inadequate framework for regulation, inadequate investment climate and poor access to technical know-how. Limited data on the availability of this biomass is also a major challenge. Improvement in research and development, raising awareness about the use of biomass, community engagement, policy support from the government and infrastructural development are some suggested recommendations to fully utilize the potential of biomass for energy production in Nigeria.
Acknowledgement: The authors from Nile University of Nigeria appreciate the availability of facilities such as the internet and access to major journal repositories to complete this study.
Funding Statement: The authors received no specific funding for this study.
Author Contributions: The authors confirm contribution to the paper as follows: study conception and design: Adekunle A. Adeleke, Nzerem Petrus, Asmau M. Yahya; data collection: Ikechukwu S. Okafor; analysis and interpretation of results: Stephen S. Emmanuel, Adebayo I. Olosho, Ademidum A. Adesibikan; draft manuscript preparation: Adekunle A. Adeleke, Asmau M. Yahya, Peter P. Ikubanni. All authors reviewed the results and approved the final version of the manuscript.
Availability of Data and Materials: Data available on request from the authors. The data that support the findings of this study are available from the corresponding author, A.A. Adeleke, upon reasonable request.
Conflicts of Interest: The authors declare that they have no conflicts of interest to report regarding the present study.
References
1. Jekayinfa, S. O., Orisaleye, J. I., Pecenka, R. (2020). An assessment of potential resources for biomass energy in Nigeria. Resources, 9(8), 92. [Google Scholar]
2. Sokan-Adeaga, A. A., Ana, G. R. E. E. (2015). A comprehensive review of biomass resources and biofuel production in Nigeria: Potential and prospects. Reviews on Environmental Health, 30(3), 143–162. [Google Scholar] [PubMed]
3. Opeyemi, B. M. (2021). Path to sustainable energy consumption: The possibility of substituting renewable energy for non-renewable energy. Energy, 228(7), 120519. [Google Scholar]
4. Adeleke, A. A., Odusote, J. K., Lasode, O. A., Ikubanni, P. P., Madhurai, M. et al. (2021). Evaluation of thermal decomposition characteristics and kinetic parameters of melina wood. Biofuels, 13(1), 117–123. [Google Scholar]
5. Akinrinola, F. S., Darvell, L. I., Jones, J. M., Williams, A., Fuwape, J. A. (2014). Characterization of selected Nigerian biomass for combustion and pyrolysis applications. Energy & Fuels, 28(6), 3821–3832. [Google Scholar]
6. Raina, S., Singh, H. M., Kothari, R., Singh, A., Allen, T. et al. (2022). Biomass to energy: Scope, challenges and applications. In: Kothari, R., Singh, A., Arora, N. K. (Eds.Biomass, bioenergy & bioeconomy, pp. 3–24. Singapore: Springer. [Google Scholar]
7. Owebor, K., Diemuodeke, E. O., Briggs, T. A., Imran, M. (2021). Power situation and renewable energy potentials in Nigeria—A case for integrated multi-generation technology. Renewable Energy, 177(2), 773–796. [Google Scholar]
8. Simonyan, K. J., Fasina, O. (2013). Biomass resources and bioenergy potentials in Nigeria. African Journal of Agricultural Research, 8, 4975–4989. [Google Scholar]
9. Sharma, V., Tsai, M. L., Nargotra, P., Chen, C. W., Kuo, C. H. et al. (2022). Agro-industrial food waste as a low-cost substrate for sustainable production of industrial enzymes: A critical review. Catalysts, 12(11), 1373. [Google Scholar]
10. Akogun, O. A., Waheed, M. A. (2019). Property upgrades of some raw Nigerian biomass through torrefaction pre-treatment—A review. IOP Conference Series: Journal of Physics, 1378(3), 32026. [Google Scholar]
11. Emmanuel, S. S., Olawoyin, C. O., Ayodele, I. D., Oluwole, O. J. (2023). Emerging nanosemiconductors for photocatalytic degradation of mono-aromatic volatile organic compounds (BTEXA pragmatic review. Journal of Organometallic Chemistry, 996, 122767. [Google Scholar]
12. Emmanuel, S. S., Adesibikan, A. A., Saliu, O. D., Opatola, E. A. (2023). Greenly biosynthesized bimetallic nanoparticles for ecofriendly degradation of notorious dye pollutants: A review. Plant Nano Biology, 3(2), 100024. [Google Scholar]
13. Agba, A. M. O., Ushie, M. E., Abam, F. I., Agba, M. S., Okoro, J. (2010). Developing the biofuel industry for effective rural transformation in Nigeria. European Journal of Scientific Research, 40, 441–449. [Google Scholar]
14. Olanrewaju, F. O., Andrews, G. E., Li, H., Phylaktou, H. N. (2019). Bioenergy potential in Nigeria. Chemical Engineering Transactions, 74, 61–66. [Google Scholar]
15. Mshelia, R. B. (2021). Assessment of renewable energy potentials of the northeast geopolitical region of Nigeria. Renewable Energy Sources Energy Policy and Energy Management, 2(2), 24–38. [Google Scholar]
16. Ezealigo, U. S., Otoijamun, I., Onwualu, A. P. (2021). Electricity and biofuel production from biomass in Nigeria: Prospects, challenges and way forward. IOP Conference Series: Earth and Environmental Science, 730(1), 12035. [Google Scholar]
17. Ben-Iwo, J., Manovic, V., Longhurst, P. (2016). Biomass resources and biofuels potential for the production of transportation fuels in Nigeria. Renewable and Sustainable Energy Reviews, 63, 172–192. [Google Scholar]
18. Lee, K. T., Ofori-Boateng, C. (2013). Economic sustainability assessment of biofuels production from oil palm biomass. In: Lee, K. T., Ofori-Boateng, C. (Eds.Sustainability of biofuel production from oil palm biomass, pp. 189–215. Singapore: Springer. [Google Scholar]
19. Giwa, A., Alabi, A., Yusuf, A., Olukan, T. (2017). A comprehensive review on biomass and solar energy for sustainable energy generation in Nigeria. Renewable and Sustainable Energy Reviews, 69, 620–641. [Google Scholar]
20. Cai, J., He, Y., Yu, X., Banks, S. W., Yang, Y. et al. (2017). Review of physicochemical properties and analytical characterization of lignocellulosic biomass. Renewable and Sustainable Energy Reviews, 76, 309–322. [Google Scholar]
21. Voloshin, R. A., Rodionova, M. V., Zharmukhamedov, S. K., Veziroglu, T. N., Allakhverdiev, S. I. (2016). Biofuel production from plant and algal biomass. International Journal of Hydrogen Energy, 41(39), 17257–17273. [Google Scholar]
22. Tursi, A. (2019). A review on biomass: Importance, chemistry, classification, and conversion. Biofuel Research Journal, 6(2), 962–979. [Google Scholar]
23. Ezugwu, C. N. (2015). Renewable energy resources in Nigeria: Sources, problems and prospects. Journal of Clean Energy Technologies, 3(1), 68–71. [Google Scholar]
24. Kaur, M., Kumar, M., Sachdeva, S., Puri, S. K. (2018). Aquatic weeds as the next generation feedstock for sustainable bioenergy production. Bioresource Technology, 251(4), 390–402. [Google Scholar] [PubMed]
25. Adewuyi, A. (2020). Challenges and prospects of renewable energy in Nigeria: A case of bioethanol and biodiesel production. Energy Reports, 6(24), 77–88. [Google Scholar]
26. Elehinafe, F. B., Okedere, O. B., Fakinle, B. S., Sonibare, J. A. (2017). Assessment of sawdust of different wood species in Southwestern Nigeria as source of energy. Energy Sources, Part A: Recovery, Utilization, and Environmental Effects, 39(18), 1901–1905. [Google Scholar]
27. Umar, H. A., Sulaiman, S. A., Ahmad, R. K., Tamili, S. N. (2020). Characterisation of oil palm trunk and frond as fuel for biomass thermochemical. IOP Conference Series: Materials Science and Engineering, 863(1), 12011. [Google Scholar]
28. Lin, F., Waters, C. L., Mallinson, R. G., Lobban, L. L., Bartley, L. E. (2015). Relationships between biomass composition and liquid products formed via pyrolysis. Frontiers in Energy Research, 3, 45. [Google Scholar]
29. Banerjee, A., Bansal, N., Kumar, J., Bhaskar, T., Ray, A. et al. (2021). Characterization of the de-oiled yeast biomass for plausible value mapping in a biorefinery perspective. Bioresource Technology, 337(79), 125422. [Google Scholar] [PubMed]
30. Balogun, K., Lasode, M., Onokwai, A. O., Ezugwu, C. A., Olayanju, T. M. A. et al. (2019). Elemental analysis and combustion characteristics evaluation of Nigerian biomass resources. International Journal of Mechanical Engineering and Technology, 10(2), 1522–1527. [Google Scholar]
31. Mythili, R., Venkatachalam, P., Subramanian, P., Uma, D. (2013). Characterization of bioresidues for biooil production through pyrolysis. Bioresource Technology, 138(3), 71–78. [Google Scholar] [PubMed]
32. Chang, S. H. (2014). An overview of empty fruit bunch from oil palm as feedstock for bio-oil production. Biomass and Bioenergy, 62(9), 174–181. [Google Scholar]
33. Eze-Ilochi, N. O., Oti, W. J. (2017). Characterization of selected Nigerian indigenous biomass wastes for their suitability in biofuel production. International Journal of Pharmaceutical Science Invention, 6(9), 1–8. [Google Scholar]
34. Singh, Y. D., Mahanta, P., Bora, U. (2017). Comprehensive characterization of lignocellulosic biomass through proximate, ultimate and compositional analysis for bioenergy production. Renewable Energy, 103(11), 490–500. [Google Scholar]
35. Padilla, E. R. D., Nakashima, G. T., Hansted, A. L. S., Santos, L. R. O., de Barros, J. L. et al. (2019). Thermogravimetric and FTIR analyzes of corn cob pyrolysis. Quimica Nova, 42(5), 566–569. [Google Scholar]
36. Chukwuneke, J. L., Ewulonu, M. C., Chukwujike, I. C., Okolie, P. C. (2019). Physico-chemical analysis of pyrolyzed bio-oil from swietenia macrophylla (mahogany) wood. Heliyon, 5(6), e01790. [Google Scholar] [PubMed]
37. Wang, S., Zhao, S., Uzoejinwa, B. B., Zheng, A., Wang, Q. et al. (2020). A state-of-the-art review on dual purpose seaweeds utilization for wastewater treatment and crude bio-oil production. Energy Conversion and Management, 222, 113253. [Google Scholar]
38. Awoyale, A. A., Lokhat, D. (2021). Experimental determination of the effects of pretreatment on selected Nigerian lignocellulosic biomass in bioethanol production. Scientific Reports, 11, 557. [Google Scholar] [PubMed]
39. Efetobor, U. J., Ikpeseni, S. C., Sada, S. O. (2022). Determination of proximate, ultimate and structural properties of elephant grass as biomass material for bio-oil production. Journal of Applied Science and Environmental Management, 26(12), 1903–1907. [Google Scholar]
40. Olawale, C. A., Ogunsuyi, H. O. (2020). Post-harvested plantain biomass as potential feedstock for bio-oil. International Journal of Engineering Applied Sciences and Technology, 5(5), 53–59. [Google Scholar]
41. Ahmmed, M., Halim, M., Mortuza, M., Ismail, M. (2020). Potato (Solanum tuberosum) peel waste utilization for eco-friendly bio-oil production via pyrolysis. DUJASE, 5, 25–29. [Google Scholar]
42. Anukam, A. I., Goso, B. P., Okoh, O. O., Mamphweli, S. N. (2017). Studies on characterization of corn cob for application in a gasification process for energy production. Journal of Chemistry, 2017, 1–9. [Google Scholar]
43. Ibeto, C., Anisha, M., Anyanwu, C. (2016). Evaluation of the fuel properties and pollution potentials of lignite coal and pellets of its blends with different biowastes. American Chemical Science Journal, 14(1), 1–12. [Google Scholar]
44. Shariff, A., Aziz, N. S. M., Ismail, N. I., Abdullah, N. (2016). Corn cob as a potential feedstock for slow pyrolysis of biomass. Journal of Physical Sciences, 27(2), 123–137. [Google Scholar]
45. Liu, X., Zhang, Y., Li, Z., Feng, R., Zhang, Y. (2014). Characterization of corncob-derived biochar and pyrolysis kinetics in comparison with corn stalk and sawdust. Bioresource Technology, 170, 76–82. [Google Scholar] [PubMed]
46. Onokwai, A. O., Ajisegiri, E. S. A., Okokpujie, I. P., Ibikunle, R. A., Oki, M. et al. (2022). Characterization of lignocellulose biomass based on proximate, ultimate, structural composition, and thermal analysis. Materials Today: Proceedings, 65(5), 2156–2162. [Google Scholar]
47. Sulaiman, M. A., Adetifa, B. O., Adekomaya, S. O., Lawal, N. S., Adama, O. O. (2019). Experimental characterization of maize cob and stalk based pellets for energy use. Engineering Journal, 23(6), 117–128. [Google Scholar]
48. Boluwaji, F. E., Babatunde, O. O., Abosede, O. O., Onose, M. A., Fakinle, B. S. (2019). Proximate analysis of the properties of some Southwestern Nigeria sawdust of different wood species. International Journal of Civil Engineering and Technology, 10(3), 51–59. [Google Scholar]
49. Adegoke, I. A., Ogunsanwo, O. Y., Ige, A. R. (2021). Bio-fuel properties and elemental analysis of bio-oil produced from pyrolysis of gmelina arborea. Acta Chemica Malaysia, 5(1), 38–41. [Google Scholar]
50. Mensah, I., Ahiekpor, J. C., Herold, N., Bensah, E. C., Pfriem, A. et al. (2022). Biomass and plastic co-pyrolysis for syngas production: Characterisation of celtis mildbraedii sawdust as a potential feedstock. Scientific African, 16(2), e01208. [Google Scholar]
51. Varma, A. K., Thakur, L. S., Shankar, R., Mondal, P. (2019). Pyrolysis of wood sawdust: Effects of process parameters on products yield and characterization of products. Waste Management, 89(1), 224–235. [Google Scholar] [PubMed]
52. Adekunle, A. A., Ellis-Jones, J., Ajibefun, I., Nyikal, R. A., Bangali, S. et al. (2012). Agricultural innovation in Sub-Saharan Africa: Experiences from multiple stakeholder approaches. Accra, Ghana: Forum for Agricultural Research in Africa (FARA). [Google Scholar]
53. Ndjeunga, J., Mausch, K., Simtowe, F. (2015). Assessing the effectiveness of agricultural R&D for groundnut, pearl millet, pigeonpea and sorghum in West and Central Africa and East and Southern Africa. In: Walker, T. S., Alwang, J. (Eds.Crop improvement, adoption, and impact of improved varieties in food crops in Sub-Saharan Africa, pp. 123–147. Wallingford, UK: CABI. [Google Scholar]
54. Odendo, M., Bationo, A., Kimani, S. (2011). Socio-economic contribution of legumes to livelihoods in Sub-Saharan Africa. In: Bationo, A., Waswa, B., Okeyo, J., Maina, F., Kihara, J., Mokwunye, U. (Eds.Fighting poverty in Sub-Saharan Africa: The multiple roles of legumes in integrated soil fertility management, pp. 27–46. Dordrechtp: Springer. [Google Scholar]
55. Obioha, E. E. (2009). Climate variability, environment change and food security nexus in Nigeria. Journal of Human Ecology, 26(2), 107–121. [Google Scholar]
56. Bi, H., Wang, C., Jiang, X., Jiang, C., Bao, L. et al. (2021). Thermodynamics, kinetics, gas emissions and artificial neural network modeling of co-pyrolysis of sewage sludge and peanut shell. Fuel, 284(1), 118988. [Google Scholar]
57. Arumugam, N., Biely, P., Puchart, V., Singh, S., Pillai, S. (2018). Structure of peanut shell xylan and its conversion to oligosaccharides. Process Biochemistry, 72(9), 124–129. [Google Scholar]
58. Duc, P. A., Dharanipriya, P., Velmurugan, B. K., Shanmugavadivu, M. (2019). Groundnut shell-a beneficial bio-waste. Biocatalysis and Agricultural Biotechnology, 20(15), 101206. [Google Scholar]
59. Nyachaka, C. J., Yawas, D. S., Pam, G. Y. (2013). Production and performance evaluation of bioethanol fuel from groundnuts shell waste. American Journal of Engineering Research, 2, 303–312. [Google Scholar]
60. Rapheal, I. A., Moki, E. C., Muhammad, A., Mohammed, G., Hassan, L. G. et al. (2021). Physico-chemical and combustion analyses of bio-briquettes from biochar produced from pyrolysis of groundnut shell. International Journal of Advanced Chemistry, 9(2), 74–79. [Google Scholar]
61. Varma, A. K., Singh, S., Rathore, A. K., Thakur, L. S., Shankar, R. et al. (2022). Investigation of kinetic and thermodynamic parameters for pyrolysis of peanut shell using thermogravimetric analysis. Biomass Conversion and Biorefinery, 12(11), 4877–4888. [Google Scholar]
62. Nithiya, K., Subramanian, P., Ramesh, D., Uma, D., Surendrakumar, A. (2022). Bio-oil production from groundnut shell fast pyrolysis in spouted bed reactor. Pollution Reseacrh, 41(1), 362–366. [Google Scholar]
63. Pawar, A., Panwar, N. L. (2022). Experimental investigation on biochar from groundnut shell in a continuous production system. Biomass Conversion and Biorefinery, 12(4), 1093–1103. [Google Scholar]
64. Radhakrishnan, N., Gnanamoorthi, V. (2015). Pyrolysis of groundnut shell biomass to produce bio-oil. Journal of Chemical and Pharmaceutical Sciences, 9, 34–36. [Google Scholar]
65. Narayanasamy, B., Jeyakumar, N., Balasubramanian, D. (2021). Effect of star anise as a natural antioxidant additive on the oxidation stability of lemon grass oil. Waste and Biomass Valorization, 12(6), 2983–2997. [Google Scholar]
66. Zheljazkov, V. D., Cantrell, C. L., Astatkie, T., Cannon, J. B. (2011). Lemongrass productivity, oil content, and composition as a function of nitrogen, sulfur, and harvest time. Agronomy Journal, 103(3), 805–812. [Google Scholar]
67. Madhu, P., Stephen Livingston, T., Manickam, I. N. (2017). Fixed bed pyrolysis of lemongrass (Cymbopogon flexuosusBio-oil production and characterization. Energy Sources, Part A: Recovery, Utilization, and Environmental Effects, 39(13), 1359–1368. [Google Scholar]
68. Bhavsar, P. A., Jagadale, M. H., Khandetod, Y. P., Mohod, A. G. (2018). Proximate analysis of selected non woody biomass. International Journal of Current Microbiology and Applied Science, 7(9), 2846–2849. [Google Scholar]
69. McKendry, P. (2002). Energy production from biomass (part 2Conversion technologies. Bioresource Technology, 8(1), 47–54. [Google Scholar]
70. McKendry, P. (2002). Energy production from biomass (part 1Conversion technologies. Bioresource Technology, 83(1), 37–46. [Google Scholar] [PubMed]
71. Agbro, E. B., Ogie, N. A. (2012). A comprehensive review of biomass resources and biofuel production potential in Nigeria. Research Journal in Engineering and Applied Sciences, 1, 149–155. [Google Scholar]
72. Schlag, N., Zuzarte, F. (2008). Market barriers to clean cooking fuels in Sub-Saharan Africa: A review of literature. https://www.jstor.org/stable/pdf/resrep00344.1.pdf [Google Scholar]
73. Ali, O. J., Victor, M. A. (2013). Assessment of socio-economic factors affecting household charcoal use in Makurdi urban area of Benue State, Nigeria. Journal of Environmental Research and Management, 3, 180–188. [Google Scholar]
74. Orimoogunje, O. O. I., Asifat, J. (2015). Fuel wood consumption and species degradation in South-Western Nigeria: The ecological relevance. Journal of Landscape Ecology, 8(1), 56–68. [Google Scholar]
75. Ajibola, A. F., Raimi, M. O., Steve-Awogbami, O. C., Adeniji, A. O., Adekunle, A. P. (2020). Policy responses to addressing the issues of environmental health impacts of charcoal factory in Nigeria: Necessity today; essentiality tomorrow. Communication, Society and Media, 3(3), 1–36. [Google Scholar]
76. Buba, A., Abdu, M., Adamu, I., Jibir, A., Usman, Y. I. (2017). Socio-economic determinants of households fuel consumption in Nigeria. International Journal of Research, 5, 348–360. [Google Scholar]
77. Olorunnisola, A. O., Richards, A., Omoniyi, T. E. (2021). A review on the renewable energy from agricultural and forest residues in Nigeria. International Journal for Research and Technology, 3(1), 4–11. [Google Scholar]
78. Umar, H. A., Sulaiman, S. A., Said, M. A., Gungor, A., Ahmad, R. K. (2021). An overview of biomass conversion technologies in Nigeria. In: Sulaiman, S. A. (Ed.Clean energy opportunities in tropical countries, pp. 133–150, Singapore: Springer. [Google Scholar]
79. Limayem, A., Ricke, S. C. (2012). Lignocellulosic biomass for bioethanol production: Current perspectives, potential issues and future prospects. Progress in Energy and Combustion Science, 38(4), 449–467. [Google Scholar]
80. Devi, A., Bajar, S., Sihag, P., Sheikh, Z. U. D., Singh, A. et al. (2023). A panoramic view of technological landscape for bioethanol production from various generations of feedstocks. Bioengineered, 14(1), 81–112. [Google Scholar] [PubMed]
81. Balat, M., Balat, H., Öz, C. (2008). Progress in bioethanol processing. Progress in Energy and Combustion Science, 34(5), 551–573. [Google Scholar]
82. Zabed, H., Sahu, J. N., Suely, A., Boyce, A. N., Faruq, G. (2017). Bioethanol production from renewable sources: Current perspectives and technological progress. Renewable and Sustainable Energy Reviews, 71(32), 475–501. [Google Scholar]
83. Sarkar, N., Ghosh, S. K., Bannerjee, S., Aikat, K. (2012). Bioethanol production from agricultural wastes: An overview. Renewable Energy, 37(1), 19–27. [Google Scholar]
84. Singhania, R. R., Patel, A. K., Raj, T., Tsai, M. L., Chen, C. W. et al. (2022). Advances and challenges in biocatalysts application for high solid-loading of biomass for 2nd generation bio-ethanol production. Catalysts, 12(6), 615. [Google Scholar]
85. Vohra, M., Manwar, J., Manmode, R., Padgilwar, S., Patil, S. (2014). Bioethanol production: Feedstock and current technologies. Journal of Environmental Chemical Engineering, 2(1), 573–584. [Google Scholar]
86. Novia, N., Hasanudin, H., Hermansyah, H., Fudholi, A., Pareek, V. K. (2023). Recent advances in CFD modeling of bioethanol production processes. Renewable and Sustainable Energy Reviews, 183(18), 113522. [Google Scholar]
87. Antunes, F. A. F., Freitas, J. B. F., Prado, C. A., Castro-Alonso, M. J., Diaz-Ruiz, E. et al. (2023). Valorization of corn cobs for xylitol and bioethanol production through column reactor process. Energies, 16(13), 4841. [Google Scholar]
88. Yadav, A., Sharma, V., Tsai, M. L., Chen, C. W., Sun, P. P. et al. (2023). Development of lignocellulosic biorefineries for the sustainable production of biofuels: Towards circular bioeconomy. Bioresource Technology, 381(1), 129145. [Google Scholar] [PubMed]
89. Patil, N., Shendkar, T. G., Pardhi, A., Suthar, S. P., Patil, G. S. et al. (2023). Bioethanol production from agricultural biomass: Sources of cellulose, pretreatment methods, and future prospects. In: Pathak, P. D., Mandavgane, S. A. (Eds.Biorefinery: A sustainable approach for the production of biomaterials, biochemicals and biofuels, pp. 287–324. Singapore: Springer. [Google Scholar]
90. Robak, K., Balcerek, M. (2018). Review of second generation bioethanol production from residual biomass. Food Technology and Biotechnology, 56(2), 174–187. [Google Scholar] [PubMed]
91. Oloruntoba, A., Adekanye, P. K. (2019). Biofuel development in nigeria production and policy challenges. Journal of Energy Resesearch and Reviews, 3, 1–21. [Google Scholar]
92. Mohammed, Y. S., Mustafa, M. W., Bashir, N., Ogundola, M. A., Umar, U. (2014). Sustainable potential of bioenergy resources for distributed power generation development in Nigeria. Renewable and Sustainable Energy Reviews, 34, 361–370. [Google Scholar]
93. Ohimain, E. I. (2015). The evaluation of pioneering bioethanol projects in Nigeria following the announcement and implementation of the Nigerian biofuel policy and incentives. Energy Sources, Part B: Economics, Planning, Policy, 10(1), 51–58. [Google Scholar]
94. Ohimain, E. I. (2010). Emerging bio-ethanol projects in Nigeria: Their opportunities and challenges. Energy Policy, 38(11), 7161–7168. [Google Scholar]
95. Oyedepo, S. O., Dunmade, I. S., Adekeye, T., Attabo, A. A., Olawole, O. C. et al. (2019). Bioenergy technology development in Nigeria-pathway to sustainable energy development. International Journal of Environment and Sustainable Development, 18(2), 175–205. [Google Scholar]
96. Olanipekun, B. A., Adelakun, N. O. (2020). Assessment of renewable energy in Nigeria: Challenges and benefits. International Journal of Engineering Trends and Technology, 68(1), 64–67. [Google Scholar]
97. Aliyu, A. K., Modu, B., Tan, C. W. (2018). A review of renewable energy development in Africa: A focus in South Africa, Egypt and Nigeria. Renewable and Sustainable Energy Reviews, 81(6), 2502–2518. [Google Scholar]
98. Olujobi, O. J., Ufua, D. E., Olokundun, M., Olujobi, O. M. (2021). Conversion of organic wastes to electricity in Nigeria: Legal perspective on the challenges and prospects. International Journal of Environmental Science and Technology, 19(2), 939–950. [Google Scholar]
99. Aliyu, A. S., Deba, A. A., Saidu, H., Mohammed, I. L., Usman, M. M. (2017). Biofuel development in Nigeria: Prospect and challenges. Journal of Advanced Research in Fluid Mechanics and Thermal Sciences, 36(1), 1–9. [Google Scholar]
Cite This Article
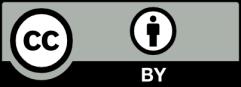