Open Access
ARTICLE
Morphological and Phylogenetic Resolution of Diplodia neojuniperi Emerging Diplodia Top Dieback of Pinus thunbergii Parl. in China
Co-Innovation Center for Sustainable Forestry in Southern China, College of Forestry, Nanjing Forestry University, Nanjing, 210037, China
* Corresponding Author: Jianren Ye. Email:
Phyton-International Journal of Experimental Botany 2022, 91(12), 2813-2825. https://doi.org/10.32604/phyton.2022.024609
Received 03 June 2022; Accepted 20 July 2022; Issue published 29 August 2022
Abstract
In Bazhong City, Sichuan Province, China, top dieback symptoms were found on many pine trees (Pinus thunbergii Parl). The tips of old needles first turned grayish-green and then developed into brown bands in the field. Phylogenetic analysis of concatenated ITS and EF1-α indicated the pathogen of this dieback disease as Diplodia neojuniperi. Additionally, effects of temperature, pH and medium on the mycelial growth were also characterized. The most favorable temperature and pH level for mycelial growth are 25°C and 8, respectively. The optimal medium for mycelial growth is PDA medium. To our knowledge, this is the first report of D. neojuniperi causes Diplodia top dieback on Pinus thunbergii. Our results provide fundamental information for monitoring and preventing such disease in the future.Keywords
Pine needle, known as pine leaves and fur, is one of the most important by-products of pine trees. It is also the main medical component of Pinaceae plants, such as Pinus thunbergii Parl., P. massoniana Lamb. and P. koraiensis [1–3]. Besides, pine needles also contain indispensable inorganic elements for the human body, such as flavonoids, volatile oil, shikimic acid, fatty acid, phosphorus, calcium, and multiple amino acids, etc. The extraction from pine needles is a natural high-quality compound which show no negative effects on mammals [1]. Such compounds are not only avirulent but also possess immunity enhancing and many other benefits on humans like hypolipidemic, hypoglycemic, antioxidant, antiviral, antibiosis according to previous studies [4,5].
Pinus thunbergii Parl. (Japanese black pine) is a widely distributed pine species in China due to its graceful leaf shape, fast growth, and salinity tolerance, which originated from Japan and North Korea [6]. It plays essential roles in ecological restoration such as sand fixation, and environment afforestation [7]. However, in June 2020, a large area of P. thunbergii were observed to show discolored and withered needles in forests from Bazhong City, Sichuan Province, China. This unknown disease has affected the potential value of pine needles, decreased the growth of pine trees and caused serious economic and ecological losses.
Many pathogens which would cause pine wilt and death on P. thunbergii have been reported in China. The pine wood nematode could infect P. thunbergii and causing severe pine wilt disease within a very short time [8]. Matsucoccus matsumurae could destroy the inner bark tissue of P. thunbergii, and eventually make the branches soft and droop. This insect did not kill pine trees but made them more vulnerable to other pathogens and insects in order to bring significant damage to P. thunbergii [9]. Needle-shedding disease [10] of P. thunbergii was mainly caused by Lophodermium spp. which severely damaged pine needles. At the beginning of the infection, small yellow spots with brownish markings would appear, the yellowing leaves could not recover in the late stage and eventually fell off. Needle-shedding disease is extremely contagious and possesses high morbidity. However, there is hardly any infections can be found after the needles have fully grown [11]. Also, brown-spot needle blight on P. thunbergii could cause spots and chlorosis on its needles [12]. It is common to find pine needles exhibiting discoloration and wilt symptoms in the field, however, the symptoms of white P. thunbergii needles found on the forest farms from Sichuan Province are inconsistent with aforementioned descriptions.
Diplodia spp. have been found more than 1,500 species in the earth by far. They usually infect woody plants and are acknowledged as endophytes, saprophytic fungi, and pathogenic fungi [13]. Some of the Diplodia spp. are associated with leaf, stem [14] and root [15] diseases in a variety of plant hosts to cause severe forest disease. It has been affirmed that this genus can lead to leaf rotten disease on Italian cypress, dieback on Corylus heterophylla Fisch, Australia grapevines, and oak wilt disease [16]. Diplodia spp. belongs to Botryosphaeriaceae which used to be considered as a synonym of Lasiodiplodia spp. [17]. However, many subsequent studies demonstrate that there are distinct differences between Lasiodiplodia and Diplodia on both morphological and phylogenetic levels [18,19]. Thus, they should be classified into different genera.
Top dieback resulting from pine canker is a serious pine needle disease with a worldwide distribution and has more than 80 pine hosts according to reports [20]. As one of the most important limiting factors for tree production in nursery and forest environments, pine canker can induce premature needle loss and reduced photosynthetic capacity, weakening the pine tree and can even lead to its death [21]. At present, the pathogen of tree needle disease in Bazhong City remains unclear, which makes it difficult to assess the level of this disease in the area. The main goal of this study is to investigate the incidence and symptoms of top dieback disease in Bazhong, detect the causative pathogen based on classical Koch’s Law principle, determine the taxonomy of pathogen by morphological and molecular methods to fully understand the disease.
2.1 Field Surveys and Fungal Isolations, Purification and Reservation
From June to July 2020, an investigation was conducted on tree farms in Bazhong, Sichuan to describe the incidence and severity of top dieback disease on P. thunbergii. Altogether 20 needles and 3 branches with typical symptoms were collected as isolation materials, disease/health junction parts were cut into 0.5 cm tissue blocks with sterile blades. Tissue blocks were then rinsed in 1.5% sodium hypochlorite solution for 90 s, and washed in sterile water for 3 times. Sterilized tissues (5 tissues per dish) were cultured on potato dextrose agar (PDA) medium and incubated in a dark incubator at 25°C.
Two days after incubation, colonies were selected based on their morphology, color, and growth rate. Mycelium was picked with a sterile toothpick and placed on a new PDA medium. This procedure was repeated until a single colony was obtained. After cultivating the purified colonies in the incubator, the fungal spores were washed off with sterile water to discard mycelium. The spore solution and 50% glycerol were added in a 1:1 ratio to 2 mL sterile centrifuge tubes and stored at −20°C. The filter paper preservation method was used for non-spore producing or difficult to produce fungi [22].
2.2 Pathogenicity Test and Pathogen Re-Isolation, Determination and Description
In order to determine the pathogen fungus on black pine, three-year-old P. thunbergii seedlings were used for pathogenicity test in the greenhouse of at Nanjing Forestry University. Mycelium cultured for 7 days were inoculated on black pine needles, and covered with cotton to maintain moisture. PDA medium were also inoculated on black pine trees and served as control sample. The inoculation experiments were repeated 3 times for every putative pathogenic fungus and symptom was recorded after approximately 15 days post-inoculation.
Photographs of inoculated plants which showed similar symptoms to those found in the field were taken and pathogens of diseased plants were re-isolated and observed. Strains with similar morphology were inoculated again as described above to determine the pathogen. After finishing the Koch’s postulate, the pathogen was cultured on PDA medium in a dark incubator at 25°C for 5 days to measure and observe its hyphae growth, colony and pigment [23]. The pathogen conidia and their characteristics (shape, color, size, and with or without diaphragm) were recorded. Sporulating colony on PNA was first cut into section by LEICA CM1900 then recorded by SteREO Discovery.V20 (ZEISS). The pictures of spores, sporulating colony and conidiogenous cells were taken by Axio Imager A2m microscope (Zeiss). The method reported by Úrbez-Torres was applied to generate spores when it cannot be observed on normal medium [24].
2.3 PCR Amplification, and Phylogenetic Analysis of Pathogenic Fungi
Cetyltrimethylammonium bromide (CTAB) was used to extract the DNA of aforementioned putative pathogens [25]. Oligonucleotide primers ITS1 and ITS4, and EF1-728F and EF1-986R were used to amplify the internal transcribed spacer (ITS) region and the translation elongation factor (EF1-α) gene, respectively. The PCR reaction conditions for ITS and EF1-α were conducted as described by Úrbez-Torres [24]. For each sequence, the amplified product was detected by 1% agarose gel. Primer synthesis and nucleic acid sequencing of PCR products were carried out by Nanjing Shenggong Biotechnology Corporation (Jiangsu, China).
The sequences were submitted to GenBank via Bankit to obtain accession numbers. The ITS, and EF1-α sequences of the pathogens were aligned with Blast (http://www.ncbi.nlm.nih.gov) to perform preliminary classification. Next, the sequences of Diplodia spp. used for phylogeny analysis were downloaded from NCBI website as reported in relevant study (Table 1) [25]. Downloaded sequences were aligned, spliced, and connected to generate new tandem sequences via BioEdit [26]. A multilocus phylogenetic tree was constructed using maximum likehood method with 1000 bootstraps by Mega8.0 based on ITS, and EF1-α sequences [27,28].
2.4 Biological Characterization Analysis
The identified pathogen was first cultured and cut into 6 mm diameter blocks with sterilized perforator. The blocks were inoculated into different medium under various pH and temperature to perform single factor tests (Table 2) with three replicates. After cultivating for 8 days, the mycelium diameters were measured. All statistical analyses were conducted by SPSS v.17.5 and the histograms were plotted by Origin 8.0 (https://www.originlab.com).
3.1 Field Studies on the Progression and Characteristics of the Disease
A large amount of yellowish and wilt symptoms on black pine needles were observed on a tree farm from Bazhong City, Sichuan Province in June 2020. The initial observation of black pine discoloration and wilt was around mid-May. The disease incidence is less than 30% while the severity of the disease is high. The disease incidence reached its peak in July and August when the temperature rose and the rainy season began, sometimes the peak period would last till September. The disease progress is rapid. Once the outbreak has occurred, it can infect pines at different ages, and result in wilt and death of pine needles. In the early stage of the disease, black pine needles developed sporadic brown spots. In the latter stage, infected black pine needles turned brown and died eventually (Fig. 1A).
Figure 1: Symptoms of Diplodia neojuniperi. on Pinus thunbergii. (A) Diseased branches in the field. (B) No disease symptoms were observed on control plants. (C) Inoculation on the stem of Pinus thunbergii seedlings with myceliums
3.2 Results of Fungal Isolation Diversity and Molecular Identification
A total of 6 fungal isolates were retrieved from fruiting bodies of the symptomatic needles from above-mentioned Pinus species. The purified strains belonged to the genera Pseudocercospora, Phyllosticta, Diplodia, Alternaria, and Fusarium respectively. ITS and EF1-α regions of those 6 isolated strains were amplified for better identification. The identification results of 6 purified fungi were Pseudocercospora pini-densiflorae, Phyllosticta capitalensis, Diplodia neojuniperi, Alternaria alternata, Fusarium fujikuroi and F. proliferatum (Fig. 2 and Table 3).
Figure 2: 6 purified fungal isolates of the symptomatic needles form Pinus species. (A) Pseudocercospora pini-densiflorae. (B) Phyllosticta capitalensis. (C) Diplodia neojuniperi. (D) Alternaria alternata. (E) Fusarium fujikuroi. (F) F. proliferatum
3.3 Pathogenicity Test, Morphological and Phylogenetic Analysis of the Pathogenic Fungus
Ten days after inoculation with Diplodia neojuniperi strains (SC04), Pinus thunbergii needles had leaf chlorosis. One week later, the needles first turned brown, then wilting, and dead. The symptoms were consistent with those in the field, and the incidence of disease was 100% (Figs. 1B and 1C). On contrary, there is no change in the branches that were inoculated with other purified fungus. Since Diplodia neojuniperi was isolated from diseased needles and branches, the Koch’s Law test was completed after inoculation experiments and SC04 strain was selected for downstream observation and analysis. Colony was white on PDA at first, then turned dark brown after 7 days and the outer edge was beige (Fig. 3A). The colony of Diplodia neojuniperi can sporulate on PNA medium (Figs. 3B and 3C). The conidia (Figs. 3E and 3F) were transparent, and their length were 7–12.5 μm × 2.5–4.5 μm (mean = 9.5 × 3.6 μm, n = 20). The mature conidium (Fig. 3G) was brown with one septate, its central part has constriction. It has rounded ends and its length was 21–27 μm × 10–15 μm (mean = 23.6 × 12.6 μm, n = 20).
Figure 3: Morphological characteristics of Diplodia neojuniperi. (A) Colony on potato dextrose agar in 9-cm Petri dish incubated at 25°C. Bar =1 cm. (B) Colony sporulating on PNA. Bar = 1000 μm. (C) Vertical section through conidium. Bar = 50 μm. (D) Conidiogenous cells. Bar = 10 μm. (E, F) Hyaline conidia. Bar = 10 μm. (G) Mature, 1-septate, brown conidium. Bar = 20 μm
According to the phylogenetic tree (Fig. 4) construction of SC04, the ITS and EF1-α sequences of SC04 matched Diplodia neojuniperi at a high level. The bootstrap support of strain SC04 and Diplodia neojuniperi is 96%. The ITS and EF1-α sequences (Accession Nos. ON340772 and ON364524) were deposited in GenBank.
Figure 4: Phylogenetic analysis of SC04 using ITS and EF1-α
3.4 Effects of pH, Temperature and Medium on Mycelial Growth
We evaluate the growth rate of SC04 under different pH, temperature and culture medium to investigate its optimal growth condition. Strain SC04 can grow in the range of pH 4 to 11. The most suitable pH level for strain SC04 is 8, the growth of SC04 was significantly inhibited when pH dropped less than 4 (Fig. 5). The strain SC04 can grow in a range of 10°C to 30°C (Fig. 6). The most favorable temperature is 25°C condition and the colony diameter reached 9.0 cm within 8 days, followed by 20°C. Among the different medium we used in this study, the optimal medium for mycelial growth of strain SC04 is PDA medium followed by OA medium, MEA medium, PSA medium, and Czapek medium (Fig. 7). On PDA medium, the mycelium diameter of SC04 reached 9.0 cm after 8 days with a thick and vigor appearance. The mycelium was green on MEA medium and OA medium, and it can hardly grow on Czapek medium.
Figure 5: Effects of different PH on mycelial growth of strain SC04 and colonies of SC04 on culture medium with different pH levels. (A) Histogram of SC04 growth condition with different pH levels. (B) Mycelial growth of strain SC04 under pH4, (C) pH5, (D) pH6, (E) pH7, (F) pH8, (G) pH9 and (H) pH10
Figure 6: Effects of different temperatures (°C) on mycelial growth of strain SC04 and colonies of strain SC04 under different temperature conditions. (A) Histogram of SC04 growth condition with different temperature. (B) Mycelial growth of strain SC04 under 5°C, (C) 10°C, (D) 15°C, (E) 20°C, (F) 25°C, (G) 30°C and (H) 35°C
Figure 7: Mycelial growth of strain SC04 and colonies of strain SC04 on different medium. (A) Growth rate of mycelia on different medium. (B) Mycelial growth of strain SC04 on PDA, (C) MEA, (D) OA, (E) Czapek and (F) PSA
To our knowledge, this is the first report of Diplodia dieback on P. thunbergii caused by Diplodia neojuniperi in China. Needle wilt caused by this fungus greatly affected the health of the pine forest. The previous field survey of our study has found that branch injury and needle wilt caused by D. neojuniperi have a long-term influence on the vitality of pine forests and can bring death to heavily infected pine trees within two years. The mortality resulting from this disease depends entirely on the extent to which the crown is affected by the infection but has nothing to do with the growth of host trees prior to initial infection. Besides, the incidence of the disease is also not related to the growth status of the tree. The pathogenic mechanism behind the death of pine trees caused by D. neojuniperi is unclear. Further study is required to comprehend the potential reason for the death of P. thunbergii infected by D. neojuniperi.
Diplodia top dieback has a direct impact on the maturation of pine trees [29], the defoliation caused by such disease are much harder to recover compared with similar symptom caused by defoliator [30]. The mechanism of the branches necrosis and needle defoliation caused by Diplodia top dieback remains unsolved. Oliva suggested the consumption of huge nutrition to recover the vitality of pine needles may responsible for the death of pine trees [31]. The damage or defoliation of needles directly affects the carbon uptake and photosynthetic efficiency of pine tree, which will further inhibit the absorption of water and nutrient and facilitate the development of Diplodia top dieback disease [32].
Here, Diplodia neojuniperi is the first report to cause top dieback disease on pine trees, while D. sapinea [33], D. pinea [34], D. scrobiculata [35], and D. seriata [36] are other previously discovered pathogens to cause similar diseases. Diplodia spp. have various ways to accomplish overwintering, it can survive on leaves, shoots, dead branches, and seeds. Most of Diplodia spp. have latent infection phenomenon which make it hard to prevent [37,38]. The initial infection sources were mainly from the conidium of infected tree. The conidium were spread by wind and rain to directly invade shoots and tender needles, it can also penetrates needles, shoots, tips, and branches via wounds and stomata [39,40]. The incubation period of Diplodia top dieback ranges from 7 to 14 days, its propagative period lasts from 23 to 28 days. Spore dispersion starts in early May while disease begins in Mid-June, reaches its peak from late July to late August and ends in Mid-September. Effective prevention method can be made based on the infection cycle of Diplodia spp. to control the Diplodia top dieback disease in Bazhong, Sichuan [41,42].
Changes in weather and climate may have significant influence on the occurrence and development of Diplodia top dieback caused by D. neojuniperi. The spring temperature has gradually increased for decades in Bazhong City, Sichuan Province [43–45]. Such climate changes may have important effects on seasonal precipitation and imbalanced the environmental conditions of pine forests in Bazhong City. Consequently, it will be possible to find new diseases on pine trees in those areas. These conclusions emphasize that change s of environmental factors like increasement of temperature [35] and aridity [27] could affect the growth and dispersal of various fungi in forests. Consequently, the early detection of novel pathogen D. neojuniperi on pine trees in this study provides fundamental information to better control and prevent such top dieback disease.
Acknowledgement: We thank Dr. Min Li for his valuable suggestions on revising the manuscript.
Authorship: The authors confirm contribution to the paper as follows: study conception and design: Xiaolei Ding and Jianren Ye; data collection: Ruiwen Zhao and Sixi Lin; analysis and interpretation of results: Xiaolei Ding and Ruiwen Zhao; draft manuscript preparation: Xiaolei Ding and Ruiwen Zhao. All authors reviewed the results and approved the final version of the manuscript.
Funding Statement: This project is supported by the National Natural Science Foundation of China 31800543 (X.D).
Conflicts of Interest: The authors declare that they have no conflicts of interest to report regarding the present study.
References
1. Choi, M., Kim, D. H., Kim, J. H., Kim, S. H. (1999). Short-term oral toxicity lest of the pine needle extracts in rat. Korean Journal of Food Science and Technology, 31(5), 1401–1404. [Google Scholar]
2. Jeong, M. S., Park, S., Han, E. J., Park, S. Y., Kim, M. J. et al. (2020). Pinus thunbergii PARL leaf protects against alcohol-induced liver disease by enhancing antioxidant defense mechanism in BALB/c mice. Journal of Functional Foods, 73(7), 104116. DOI 10.1016/j.jff.2020.104116. [Google Scholar] [CrossRef]
3. Kim, Y. M., Jeong, Y. K., Wang, M. H., Lee, W. Y., Rhee, H. I. (2005). Inhibitory effect of pine extract on α-glucosidase activity and postprandial hyperglycemia. Nutrition, 21(6), 756–761. DOI 10.1016/j.nut.2004.10.014. [Google Scholar] [CrossRef]
4. Jeon, J. R., Kim, J. Y. (2006). Effects of pine needle extract on differentiation of 3T3-L1 preadipocytes and obesity in high-fat diet fed rats. Biological and Pharmaceutical Bulletin, 29(10), 2111–2115. DOI 10.1248/bpb.29.2111. [Google Scholar] [CrossRef]
5. Park, Y. S., Jeon, M. H., Hwang, H. J., Park, M. R., Lee, S. H. et al. (2011). Antioxidant activity and analysis of proanthocyanidins from pine (Pinus densiflora) needles. Nutrition Research and Practice, 5(4), 281–287. DOI 10.4162/nrp.2011.5.4.281. [Google Scholar] [CrossRef]
6. Yang, H., Wang, Z., Song, W., Zhao, Z., Zhao, Y. (2021). Isolation of proanthocyanidins from Pinus thunbergii needles and tyrosinase inhibition activity. Process Biochemistry, 100(5), 245–251. DOI 10.1016/j.procbio.2020.10.003. [Google Scholar] [CrossRef]
7. Tanaka, S., Tomita, R., Saijo, H., Takahashi, K., Ashitani, T. (2020). Growth-inhibitory activity of components in Cryptomeria japonica leaves against Robinia pseudoacacia. Journal of Forest Research, 1–6(3), 192–197. DOI 10.1080/13416979.2020.1747150. [Google Scholar] [CrossRef]
8. Li, H., Shen, P., Fu, P., Lin, M., Moens, M. (2007). Characteristics of the emergence of Monochamus alternatus, the vector of Bursaphelenchus xylophilus (Nematoda: Aphelenchoididaefrom Pinus thunbergii logs in Nanjing, China, and of the transmission of the nematodes through feeding wounds. Nematology, 9(6), 807–816. DOI 10.1163/156854107782331234. [Google Scholar] [CrossRef]
9. Liu, W., Xie, Y., Dong, J., Xue, J., Zhang, Y. et al. (2014). Pathogenicity of three entomopathogenic fungi to Matsucoccus matsumurae. PLoS One, 9(7), e103350. DOI 10.1371/journal.pone.0103350. [Google Scholar] [CrossRef]
10. Hirose, D., Osono, T. (2006). Development and seasonal variations of Lophodermium populations on Pinus thunbergii needle litter. Mycoscience, 47(5), 242–247. DOI 10.1007/S10267-006-0299-3. [Google Scholar] [CrossRef]
11. Hata, K., Futai, K., Tsuda, M. (1998). Seasonal and needle age-dependent changes of the endophytic mycobiota in Pinus thunbergii and Pinus densiflora needles. Canadian Journal of Botany, 76(2), 245–250. [Google Scholar]
12. Li, C. D., Zhu, X. Q., Han, Z. M. (1986). Investigation on brown-spot needle blight of pines in China. Journal of Nanjing Forestry University, 2, 11–18. [Google Scholar]
13. He, D., Ma, R., Li, Y., Yang, X., Wang, L. (2017). Chemical constituents of the twigs of Caesalpinia mimosoides. Chemistry of Natural Compounds, 53(3), 545–547. [Google Scholar]
14. Úrbez-Torres, J., Leavitt, G., Guerrero, J., Guevara, J., Gubler, W. (2008). Identification and pathogenicity of Lasiodiplodia theobromae and Diplodia seriata, the causal agents of bot canker disease of grapevines in Mexico. Plant Disease, 92(4), 519–529. [Google Scholar]
15. Paulpietersburg, N. (1980). Association of Diplodia pinea with a root disease of pines in South Africa. Plant Disease, 64(2), 221. [Google Scholar]
16. Bi, D., Xia, G., Li, Y., Liang, X., Zhang, L. et al. (2018). Two new cassane diterpene lactams from the fruits of Caesalpinia mimosoides Lam. Natural Product Research, 32(8), 875–879. [Google Scholar]
17. Denman, S., Crous, P. W., Taylor, J. E., Kang, J. C., Pascoe, I. et al. (2000). An overview of the taxonomic history of Botryosphaeria and a re-evaluation of its anamorphs based on morphology and ITS rDNA phylogeny. Studies in Mycology, 45, 129–140. [Google Scholar]
18. Phillips, A., Alves, A., Pennycook, S., Johnston, P., Ramaley, A. et al. (2008). Resolving the phylogenetic and taxonomic status of dark-spored teleomorph genera in the Botryosphaeriaceae. Persoonia: Molecular Phylogeny and Evolution of Fungi, 21, 29–55. [Google Scholar]
19. Slippers, B., Crous, P. W., Denman, S., Coutinho, T. A., Wingfield, B. D. et al. (2004). Combined multiple gene genealogies and phenotypic characters differentiate several species previously identified as Botryosphaeria dothidea. Mycologia, 96(1), 83–101. [Google Scholar]
20. Zhou, S., Stanosz, G. R. (2001). Stanosz, Relationships among Botryosphaeria species and associated anamorphic fungi inferred from the analyses of ITS and 5.8S rDNA sequences. Mycologia, 93(3), 516–527. [Google Scholar]
21. Watt, M. S., Kriticos, D. J., Alcaraz, S., Brown, A. V., Leriche, A. (2009). The hosts and potential geographic range of Dothistroma needle blight. Forest Ecology and Management, 257(6), 1505–1519. DOI 10.1016/j.foreco.2008.12.026. [Google Scholar] [CrossRef]
22. Cerqueira, A., Alves, A., Berenguer, H., Correia, B., Gómez-Cadenas, A. et al. (2017). Phosphite shifts physiological and hormonal profile of Monterey pine and delays Fusarium circinatum progression. Plant Physiology and Biochemistry, 114(532), 88–99. DOI 10.1016/j.plaphy.2017.02.020. [Google Scholar] [CrossRef]
23. Thomasen, H., Pauklin, M., Noelle, B., Geerling, G., Vetter, J. et al. (2011). The effect of long-term storage on the biological and histological properties of cryopreserved amniotic membrane. Current Eye Research, 36(3), 247–255. DOI 10.3109/02713683.2010.542267. [Google Scholar] [CrossRef]
24. Úrbez-Torres, J., Peduto, F., Vossen, P., Krueger, W., Gubler, W. (2013). Olive twig and branch dieback: Etiology, incidence, and distribution in California. Plant Disease, 97(2), 231–244. DOI 10.1094/PDIS-04-12-0390-RE. [Google Scholar] [CrossRef]
25. Úrbez-Torres, J. R., Leavitt, G. M., Voegel, T. M., Gubler, W. (2006). Identification and distribution of Botryosphaeria spp. associated with grapevine cankers in California. Plant Disease, 90(12), 1490–1503. DOI 10.1094/PD-90-1490. [Google Scholar] [CrossRef]
26. Porebski, S., Bailey, L. G., Baum, B. R. (1997). Modification of a CTAB DNA extraction protocol for plants containing high polysaccharide and polyphenol components. Plant Molecular Biology Reporter, 15(1), 8–15. [Google Scholar]
27. Hall, T. A. (1999). BioEdit: A user-friendly biological sequence alignment editor and analysis. Nucleic Acids Symposium Series, 41, 95–98. [Google Scholar]
28. Tamura, K., Peterson, D., Peterson, N., Stecher, G., Nei, M. et al. (2011). MEGA5: Molecular evolutionary genetics analysis using maximum likelihood, evolutionary distance, and maximum parsimony methods. Molecular Biology and Evolution, 28(10), 2731–2739. [Google Scholar]
29. Saitou, N., Nei, M. (1987). The neighbor-joining method: A new method for reconstructing phylogenetic trees. Molecular Biology and Evolution, 4(4), 406–425. [Google Scholar]
30. Brodde, L., Adamson, K., Julio Camarero, J., Castaño, C., Drenkhan, R. et al. (2019). Diplodia tip blight on its way to the North: Drivers of disease emergence in Northern Europe. Frontiers in Plant Science, 9, 1818. [Google Scholar]
31. Jacquet, J. S., Orazio, C., Jactel, H. (2012). Defoliation by processionary moth significantly reduces tree growth: A quantitative review. Annals of Forest Science, 69(8), 857–866. DOI 10.1007/s13595-012-0209-0. [Google Scholar] [CrossRef]
32. Oliva, J., Stenlid, J., Martínez-Vilalta, J. (2014). The effect of fungal pathogens on the water and carbon economy of trees: Implications for drought-induced mortality. New Phytologist, 203(4), 1028–1035. DOI 10.1111/nph.12857. [Google Scholar] [CrossRef]
33. Castaño, C., Camarero, J. J., Zas, R., Sampedro, L., Bonet, J. A. et al. (2020). Insect defoliation is linked to a decrease in soil ectomycorrhizal biomass and shifts in needle endophytic communities. Tree Physiology, 40(12), 1712–1725. DOI 10.1093/treephys/tpaa104. [Google Scholar] [CrossRef]
34. Kaya, A. G. A., Yeltekin, Ş., JÄRVI, T. D. L., Lehtijärvi, A., Woodward, S. (2019). Severity of Diplodia shoot blight (caused by Diplodia sapinea) was greatest on Pinus sylvestris and Pinus nigra in a plantation containing five pine species. Phytopathologia Mediterranea, 58(2), 249–259. DOI 10.14601/Phytopathol_Mediter-10613. [Google Scholar] [CrossRef]
35. Flowers, J., Hartman, J., Vaillancourt, L. (2006). Histology of Diplodia pinea in diseased and latently infected Pinus nigra shoots. Forest Pathology, 36(6), 447–459. DOI 10.1111/j.1439-0329.2006.00473.x. [Google Scholar] [CrossRef]
36. Manzanos, T., Aragones, A., Iturritxa, E. (2017). Diplodia scrobiculata: A latent pathogen of Pinus radiata reported in Northern Spain. Phytopathologia Mediterranea, 56(2), 274–277. DOI 10.3389/fpls.2018.01818. [Google Scholar] [CrossRef]
37. Jiao, Z. J., Kan, Y. C., Huang, S. L. (2014). First report of dieback disease on cedars caused by Diplodia seriata in China. Plant Disease, 98(9), 1279. DOI 10.1094/PDIS-03-14-0309-PDN. [Google Scholar] [CrossRef]
38. Oblinger, B. W., Smith, D. R., Stanosz, G. R. (2011). Red pine harvest debris as a potential source of inoculum of Diplodia shoot blight pathogens. Forest Ecology and Management, 262(4), 663–670. DOI 10.1016/j.foreco.2011.04.038. [Google Scholar] [CrossRef]
39. Palmer, M., McRoberts, R., Nicholls, T. (1988). Sources of inoculum of Sphaeropsis sapinea in forest tree nurseries. Phytopathology, 78(6), 831–835. DOI 10.1094/Phyto-78-831. [Google Scholar] [CrossRef]
40. Storer, A. J., Gordon, T. R., Wood, D. L., Bonello, P. (1997). Pitch canker disease of pines: Current and future impacts. Journal of Forestry, 95(12), 21–26. [Google Scholar]
41. Stanosz, G. R., Smith, D. R., Leisso, R. (2007). Diplodia shoot blight and asymptomatic persistence of Diplodia pinea on or in stems of jack pine nursery seedlings. Forest Pathology, 37(3), 145–154. DOI 10.1111/j.1439-0329.2007.00487.x. [Google Scholar] [CrossRef]
42. Vujanovic, V., St-Arnaud, M., Neumann, P. J. (2000). Susceptibility of cones and seeds to fungal infection in a pine (Pinus spp.) collection. Forest Pathology, 30(6), 305–320. DOI 10.1046/j.1439-0329.2000.00211.x. [Google Scholar] [CrossRef]
43. Stanosz, G. R., Blodgett, J. T., Smith, D. R., Kruger, E. L. (2001). Water stress and Sphaeropsis sapinea as a latent pathogen of red pine seedlings. New Phytologist, 149(3), 531–538. DOI 10.1046/j.1469-8137.2001.00052.x. [Google Scholar] [CrossRef]
44. Chen, Q. Q., Tang, Z. Y., Yang, L., Song, F. L., Lu, H. G. (2017). Analysis of air temperature and precipitation in Sichuan. Journal of Chengdu University of Information Technology, 32(2), 200–207. [Google Scholar]
45. Maresi, G., Luchi, N., Pinzani, P., Pazzagli, M., Capretti, P. (2007). Detection of Diplodia pinea in asymptomatic pine shoots and its relation to the Normalized Insolation index. Forest Pathology, 37(4), 272–280. DOI 10.1111/j.1439-0329.2007.00506.x. [Google Scholar] [CrossRef]
Cite This Article
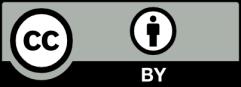