Open Access
ARTICLE
Overexpression of β-1,4-Glucanase Gene EuEG1 Improves Micrografting of Eucommia ulmoides
1 The Key Laboratory of Plant Resources Conservation and Germplasm Innovation in Mountainous Region (Ministry of Education), College of Tea Sciences, College of Life Sciences, Guizhou University, Guiyang, 550025, China
2 Guizhou Plant Conservation Technology Center, Guizhou Academy of Agricultural Sciences, Guiyang, 550006, China
3 Department of Plant Science and Landscape Architecture, University of Connecticut, Storrs, Connecticut, USA
* Corresponding Authors: Yichen Zhao. Email: ; Degang Zhao. Email:
Phyton-International Journal of Experimental Botany 2023, 92(11), 3063-3075. https://doi.org/10.32604/phyton.2023.043803
Received 12 July 2023; Accepted 25 August 2023; Issue published 24 October 2023
Abstract
Adventitious root formation poses a major constraint on the tissue culture and genetic transformation of Eucommia ulmoides. Micrografting offers a new method for the transplantation of genetic transformation, and its success depends on the formation of graft unions. This study used transgenic rootless test-tube seedlings as scions and seedlings from seed as rootstocks during micrografting to avoid the rooting issues that occur during tissue culture and to investigate the role of the EuEG1 gene in the graft healing process. We found that the EuEG1 gene is a vital regulator of graft, and its overexpression contributes to the survival of Eucommia ulmoides micrografting. The EuEG1 gene transgenic plants (TP) used as scions for micrografting presented a significantly higher survival rate than the wild type (WT) and empty vector (EV) regenerated scions. During the grafting healing process, the expression of the EuEG1 gene was higher during the period of callus proliferation, suggesting that the EuEG1 gene was involved in the graft healing process. Histological observation revealed that more calluses tissue appeared at the junction of transgenic scions, and the connection with the rootstock was stronger, which benefits wound healing. These results provide new insights into Eucommia ulmoides micrografting and indicate that the EuEG1 gene can promote wound healing and improve the micrografting survival rate.Keywords
Eucommia ulmoides Oliver (Eucommia ulmoides), a deciduous tree unique to the Eucommiaceae family, is widely used in traditional Chinese medicine [1,2]. This dioecious woody plant relies on wind-borne pollination. The differences in gene expression between male and female plants are obvious and the plants have relatively high genetic diversity [3]. However, due to the range of genetic backgrounds of the offspring, these variations in traits are not conducive to the breeding of excellent varieties. Thus, the genetic transformation technology system plays an important role in the study of Eucommia ulmoides gene function and the directional improvement of agronomic traits [4]. However, as Eucommia ulmoides is a woody plant with a long growth cycle, its tissue culture materials are slow to regenerate, and its transformation and rooting rates are extremely low [5]. This greatly limits the application of transgenic technology to Eucommia ulmoides directional improvement and the identification of gene function.
Grafting is an ancient agricultural technique which is used worldwide for the cultivation of vegetative hybrids. In this method, two segments of the plant are joined and subsequently healed to form a new singular plant. The buds or branches used as the scion are attached to an incision in the root rootstock, after which the fused plant materials heal to form a grafted plant [6]. In horticulture, grafting is widely used to improve plants’ traits and yield and to enhance biological and abiotic stress resistance [7]. Micrografting utilizes both plant tissue culture and grafting to combine the rootstocks and scions obtained from plant tissue culture in vitro [8,9]. Based on the type of scion used, micrografting methods can be divided into shoot tip grafting, microbranch grafting, callus grafting, and cell grafting [10,11], and these categories are subdivided according to the rootstock: in test tube grafting and outside test tube grafting. The scions grafted outside the test tube were obtained via tissue culture or field seedlings after disinfection, while the rootstocks were robust seedlings obtained via seed germination or cutting, which were grafted in a natural environment. Compared to traditional grafting, the scion obtained via tissue culture has a smaller size (top plant part), and it can be obtained using the same method year-round [12]. Micrografting also has several advantages, such as the ability to eliminate pathogens from plants, the mechanism of grafted union development, rejuvenate tissues, and the correlation between rootstocks and scion studies [13,14]. Furthermore, micrografting also can resolve issues with difficult rooting of tissue culture seedlings, and seasonal restrictions on the collection of scions that pose issues during traditional grafting techniques [15].
The compatibility of rootstock and scion is the main factor affecting the survival rate of grafting. The greater the compatibility, the higher the survival rate. Therefore, to further improve the success rate of grafting, it is necessary to identify additional factors that may improve the compatibility of rootstock–scion. β-1,4-glucanase is a cell wall hydrolase that hydrolyze cellulose and destroy plant cell walls [16]. β-1,4-glucanase overexpression can improve cell adhesion between grafted rootstocks and scions, promoting callus formation at the grafting interface, and improving the survival rate of grafting [17–19]. Therefore, to study the effect of β-1,4-glucanase on the grafting affinity of Eucommia ulmoides, the scion overexpressing EuEG1 gene was grafted onto the seedling rootstock, focusing on the physiological and molecular mechanisms of the wound healing process.
The seeds were collected from Eucommia ulmoides in the experimental base of Guizhou Academy of Agricultural Sciences (Guiyang, China).
The Agrobacterium tumefaciens strain LBA4404 was purchased from Transduction Biology Laboratory Co., Ltd. (Wuhan, China). pSH737 plant expression vectors were maintained by the Guizhou Institute of Agricultural Bioengineering (Guiyang, China).
2.2 Construction of Expression Vectors LBA4404 pSH737-35S-EuEG1
In this study, the pSH737-35S vector was provided by our research group, which contained the 35S promoter, GUS, a screening reporter gene; a transcription start site, and recombinase recognition sites. The EuEG1 gene containing a His tag at the 3’ was inserted into the pSH737-35S vector to construct pSH737-35S-EuEG1 using BamHI and EcoRI. Then, the plasmid containing the EuEG1 gene was extracted and transformed into Agrobacterium LBA4404-competent cells using the heat shock method.
2.3 Genetic Transformation to Cultivate Transgenic Scion
Eucommia ulmoides was transformed via a hypocotyl method mediated by Agrobacterium tumefaciens. The Eucommia ulmoides seedlings were cultivated in a germination medium for two weeks beforehand, then cut off the hypocotyls and cultured in suspension medium for 60 h. The Agrobacterium expression vector LBA4404-pSH737-35S-EuEG1 was activated and cultured for 8 h in a yeast extract peptone medium, then the bacterial cells were collected via centrifugation and used the resuspension solution resuspended the bacterial cells to OD550 was 0.3. The hypocotyls were cut into 0.5 cm pieces and infected with bacterial resuspension solution for 8 min, dried with absorbent paper, tightly inoculated on a co-culture medium, and cultured in the dark for 60 h. After that, they were transferred to a screening medium. The culture medium was changed every two weeks. After the Eucommia ulmoides resistant buds had grown to 2.5 cm, they were cut and inserted into a rejuvenation medium. The medium compositions are shown in (Table 1).
2.4 Identification of EuEG1 Transgenic Eucommia ulmoides Plants
Newly emerged leaves of resistant Eucommia ulmoides buds were transferred into the GUS tissue staining solution at 37°C for 12 h, then decolorized at 25°C with 95% ethanol for 2 d. Afterward, according to the instructions of the plant total DNA extraction kit (Yeasen, Shanghai, China), the DNA of resistant Eucommia ulmoides buds was extracted from plants stained blue by GUS. Verification was performed via PCR amplification based on the method of Dong et al. [20]. The specific verification primers 35S-F and EuEG1-V-R (Table 2), including 35S to EuEG1 common 221 bp sequence, were designed to verify the resistant Eucommia ulmoides plants. The primers GUS-F and GUS-R (Table 2) common 304 bp were designed to verify empty-vector Eucommia ulmoides plants.
One-year-old Eucommia ulmoides seedlings were planted in pots and cultivated in the greenhouse at the Guizhou Institute of Agricultural Bioengineering (China), then pruned to 30 cm above the ground to induce the growth of two-year lateral branches. Healthy lateral branches were selected as rootstocks, with a diameter of about 0.2 cm after a branch germination period of 2~4 months.
The scion and rootstock were grafted according to the cleft method. Healthy and strong lateral branches with a diameter of 0.2 cm were selected as rootstocks, and their tips were removed at approximately 2 cm above the grafting site. A 0.5 cm vertical incision was made from the middle of the rootstock. The scions needed to be acclimatized before grafting to improve the tissue culture seedlings’ ability to adapt to the changes in external conditions. Before grafting, the scions were cultured in a closed tube in an artificial climate chamber for 2 days, then the tube was opened and the culturing process continued for another 4 days. After acclimation, the base of the scion was cut into 0.5 cm long wedges (‘V’) and inserted into the grafting incision of the rootstock. The wounds were wrapped with a grafting film and fixed with a grafting clip. The grafted seedlings were cultured in an artificial climate chamber with a temperature of 24°C, 70% relative humidity, and a 14 h photoperiod with 3000 lx light intensity. There were 20 micrografting seedlings in each treatment. The survival rate was calculated after 35 days of grafting (DAG).
2.7 Anatomy Observation of the Graft Healing Process
Samples of the grafting union (0.5 cm above and below the graft region) were cut off at 3, 7, 14, and 28 DAG. They were fixed in FAA for 3 days, dehydrated in a graded ethyl alcohol series (25%, 50%, 75%, 85%, and 95%) for 30 min each, and then left in absolute ethanol for 12 h. The grafting regions were embedded in paraffin and then cut into 7 μm-thin sections by microtome. The thin sections were double stained with safranin and fast green. Observations were performed using an optical microscope [10].
2.8 Expression Analysis of EuEG1 Genes during the Healing Process of Eucommia ulmoides Grafting
Analyzed the relative expression of the EuEG1 gene in the leaves of WT/WT, EV/WT, and TP/WT scion at 0 (before grafting), 3, 7, 14, and 28 DAG. Plant total RNA extraction according to the instructions of the kit (Huayueyang, Beijing, China) and cDNA was obtained by reverse transcription kit (Applied Biosystems, Massachusetts, USA), RT-qPCR primers (Table 2) designed by (Tsingke Biotechnology Co., Ltd., Beijing, China). After that, a SYBR Green PCR Master Mix (Genstar, Shenzhen, China) was used to analyze the expression levels of the EuEG1 gene. The amplification procedure comprised the following steps: pre-denaturation at 95°C for 3 min, followed by 40 cycles of denaturation at 95°C for 10 s, and annealing at 60°C. All experiments were repeated in triplicate.
2.9 Determination of Physiological Indicators in Grafted Seedlings
The activity of superoxide dismutase (SOD), peroxidase (POD), and the malondialdehyde (MDA) content were determined in the leaves of scion according to the kit’s operating instructions t (Solarbio, Beijing, China). These tests were performed at 0 (before grafting), 3, 7, 14, and 28 DAG, and the non-grafted Eucommia ulmoides plants were used as the blank control group (CK). Each sample was analyzed in triplicate.
3.1 Screening of Transgenic Eucommia ulmoides
The plant expression vector LBA4404-pSH737-35S-EuEG1 was constructed (Fig. 1), then transformed into Eucommia ulmoides according to an Agrobacterium tumefaciens-mediated hypocotyls method (Figs. 2a–2d). The transgenic Eucommia ulmoides plants were stained blue with GUS staining solution (Fig. 3a). PCR identification of transgenic Eucommia ulmoides with a specific primer, 35S-F/EuEG1V-R (Table 2), showed bands at 221 bp in the TP seedings. EV seedings could be amplified by a 304 bp specific band with a Gus primer. There were 20 EuEG1 overexpression test-tube seedlings (Fig. 3b) and 23 pSH737 empty vector transgenic tube seedlings (Fig. 3c). Each tube seedling could provide 1~3 scions.
Figure 1: Structure of vector LBA4404-pSH737-35S-EuEG1
Figure 2: Genetic transformation and micrografting process of Eucommia ulmoides (a) Eucommia ulmoides seeding germination; (b) resistant callus induction by hypocotyls; (c) resistant bud induction; (d) resistant bud rejuvenation; (e) scion; (f) rootstock; (g) micrografting seedling; (h) graft union; Bar = 1 cm
Figure 3: Identification of transformed plants. (a) GUS staining in genetically transformed Eucommia ulmoides (left: wild type; right: transgenic); (b) PCR detection of genetic transformation of Eucommia ulmoides, M: DNA marker; WT: wild type plant; EV: empty vector plant; 1–20: transgenic plants; (c) empty vector transformation of Eucommia ulmoides, M: DNA marker, 1–23: empty-vector plant; Bar = 1 cm
3.2 Effects of Overexpression of EuEG1 Gene on the Survival Rate of Eucommia ulmoides Micrografting
First, 20 TP and 20 EV scions, then 40 WT buds with the same diameter as the transgenic scions were selected. Of these, 11 transgenic-resistant buds survived, with a survival rate of 55%. Six transgenic empty-resistant buds survived, with a survival rate of 30%, and 15 WT survived, with a survival rate of 37.5%. This shows that scion grafting of transgenic plants overexpressing the EuEG1 gene may encourage survival (Table 3).
3.3 Effect of EuEG1 Gene Overexpression on the Wound Healing of Eucommia ulmoides Micrografting
The isolation layer appeared at the beginning of the healing process of Eucommia ulmoides micrografting. On the third day of grafting, a large gap was observed between the rootstock and scion. The stained thin layer and some broken cells were visible at the wound site; this was the isolation layer (Figs. 4a, 4d, 4g) formed by the self-disintegration of injured or dead cells at the interface. The appearance of the isolation layer indicated that the wound-healing process had begun.
Figure 4: Transverse histological observation of grafting incision of Eucommia ulmoides; (a–d), the profile structure of the wild type scion/wild type rootstock at 3, 7, 14, and 28 DAG; (e–h) the profile structure of the transformed empty vector scion/wild type rootstock at 3, 7, 14 and 28 DAG; (i–l) the profile structure of the transformed EuEG1 gene scion/wild type rootstock at 3, 7, 14 and 28 DAG; St: rootstock; Sc: scion; Ca: cambium; Ci: callus; IL: isolation layer; V: catheter; Bar = 20 μm
After 7 days of grafting, the cambium cells of the rootstock were dedifferentiated into a callus under the stimulation of trauma. The callus cells accumulated outwards, which caused the isolation layer to gradually thin and break. Near the cambium of the transgenic scion, the isolation layer disappeared, and the callus proliferated in large numbers. It was connected to the callus group of the rootstock via a callus bridge (Fig. 4h). However, there was still a clear isolation layer between the empty vector scion and the wild-type scion (Figs. 4b, 4e). It is speculated that the EuEG1 gene can hydrolyze cellulose, destroy the cell wall of the isolation layer, contribute to the formation of callus, and thus improve the survival rate of micrografting.
After 14 days of grafting, the callus of the rootstock and scion began to divide in large numbers, and the cambia of the rootstock and scion were connected to form a callus bridge, which gradually filled the gap between rootstock and scion (Figs. 4c, 4f, 4i). Meanwhile, the rootstock restored the supply of water and nutrients to the scion, which is an important sign of grafting survival. Complete graft healing occurred in the TP/WT grafts, but the WT/WT and EV/WT graft unions only partially healed, with visibly deeper gaps.
After 28 days of grafting, the isolation layer at the junction of the rootstock and the scion completely disappeared, the rootstock and the scion were tightly connected, and the cambium callus differentiated into new vessels, marking the completion of the healing process: the graft had formed a new individual. Thirty-five days after grafting, the wounds had healed completely and the micrografting seedlings grew well (Figs. 5a–5c).
Figure 5: The growth of scion after 35 d of grafting; WT/WT: wild type scion/wild type rootstock; EV/WT: empty vector scion/wild type rootstock; TP/WT: transformed EuEG1gene scion/wild type rootstock; Bar = 1 cm
3.4 Effect of EuEG1 Gene on the Healing Process of Eucommia ulmoides Micrografting
The results showed that before grafting, the expression level of the EuEG1 gene in TP plants was 3.36 times higher than that in the WT and 3.58 times higher than that in the EV. Within 28 days of grafting, the relative expression of the EuEG1 gene increased initially and then decreased. The expression of the EuEG1 gene in TP/WT plants reached the highest level on the seventh day after grafting; it was 1.47 times higher than that observed before grafting. The expression of WT/WT and EV/WT reached the highest level on the 14th day after grafting, which was 2.16 and 1.98 times higher than that observed before grafting, respectively (Fig. 6). This shows that the gene is primarily involved in the wound healing stage.
Figure 6: Relative expression of EuEG1 gene in the healing process of Eucommia ulmoides micrografting at 0 (before grafting), 3, 7, 14, and 28 DAG. WT/WT: wild type scion/wild type rootstock; EV/WT: empty vector scion/wild type rootstock; TP/WT: Transformed EuEG1gene scion/wild type rootstock; The relative expression were compared with non-grafted wild type plant, Differences within the sample groups were compared according to Fisher’s exact test; **: p < 0.01; *: p < 0.05; n = 3; Error bars show standard deviations
3.5 Effects of Grafting on Protective Enzyme Activity and Malondialdehyde Content
The results showed that the SOD and POD activities in WT, EV, and TP Eucommia ulmoides increased first and subsequently decreased after grafting. Within 28 days of grafting, the SOD and POD activities of grafted plants were greater than those of non-grafted plants. On the seventh day of grafting, the SOD and POD activities of the grafted plants increased significantly, reaching 160.48, 149.63, 154.83, 3804.27, 4184.69, and 4072.7 U/g, respectively; these activities were significantly (p < 0.01) higher than the 66.945 and 2282.56 U/g recorded for non-grafted plants. They then began to decrease to the baseline level at 28 DAG (Figs. 7a, 7b). On the third day of grafting, the peroxide MDA content reached the maximum values, which were 84.49, 84.06, and 86.82 nmol/g, respectively. These values were significantly (p < 0.01) higher than those of non-grafted plants, i.e., 52.22 nmol/g. After that, they began to decrease to the baseline level at 14 DAG (Fig. 7c). It was hypothesized that graft improved the plants’ ability to scavenge reactive oxygen species, increased the anti-adversity ability of Eucommia ulmoides, and rapidly repaired the cell damage caused by grafting.
Figure 7: Physiological indices of Eucommia ulmoides micrografting at 0 (before grafting), 3, 7, 14, and 28 DAG. (a) POD activities; (b) SOD activities; (c) MDA content; WT/WT: wild type scion/wild type rootstock; EV/WT: empty vector scion/wild type rootstock; TP/WT: transformed EuEG1 gene scion/wild type rootstock; CK: non-grafted wild type plants; Differences within the sample groups were compared according to Fisher’s exact test; **: p < 0.01; *: p < 0.05; n = 3; Error bars show standard deviations
Micrografting is widely used for both agricultural and scientific applications. In this study, the hypocotyl explants of Eucommia ulmoides were used for plant tissue culture and genetic transformation. The technical system of scion grafting on seedling rootstocks was established via callus induction, regeneration, proliferation, scion acclimatization, and micrografting. Additionally, the results proved the EuEG1 gene can improve the micrografting survival rate of Eucommia ulmoides.
The key essence of the grafting healing process is the callus generation, proliferation, connection, healing, vascular bundle formation, the differentiation between rootstock and scion, and finally combination into a complete individual [21]. The generation of callus is an important anatomical feature in the early stages of graft development, establishing a callus bridge that leads to the initial adhesion of the rootstock and the scion. The callus bridge is the main pathway through which the rootstock provides water and nutrients to the scion in the early stage of grafting, which is crucial to the survival of the graft [22]. Notaguchi et al. [17] identified a key protein-β-1,4-glucanase that plays an important role in the grafting between Nicotiana benthamiana, Arabidopsis, and other plants. They observed that over-expressed NbGH9B3 under the control of a wound-induced RAP2.6 promoter can improve the success of micrografting in Arabidopsis. Within 7 days after grafting, the expression of the NbGH9B3 gene encoding β-1,4-glucanase continued to increase with the grafting time [16]. β-1,4-glucanase could accelerate wound healing through a series of physiological and molecular events, such as various phytohormones, stresses, light, and cell cycle response [23]. In this study, the scions’ overexpression of the EuEG1 gene could improve the micrografting survival rate, which indicated that the EuEG1 gene may be involved in the micrografting of Eucommia ulmoides. In the process of wound healing, it was found that the expression of the EuEG1 gene was higher during days 7 to 14, and the callus proliferated in large numbers at the wound site in this period. Additionally, as the wound healed, the expression of the EuEG1 gene began to decrease. Thus, it can be inferred that this gene is mainly involved in the callus proliferation stage. Through histological section observation, it was found that for the 14 DAG, compared with the WT/WT and EV/WT plants, the gaps of the TP/WT disappeared earlier, and more calluses were produced. The connection between the rootstock and the scion was stronger, assisting with the material exchange between the scions and rootstock. The rapid formation of callus was a critical factor in the fast graft healing process [24]. During successful grafting, a large number of calluses with regular structure appeared near the cambium in the early stage, and then the callus differentiated to form new microtubules. In contrast, during the failed grafting, only a small number of calluses formed, and scions were at risk of dying due to a water shortage. Weeks after grafting, incorrectly connected vascular bundles and insufficient catheters were observed in incompatible grafting, and it was determined that intermittent transport of microtubules would lead to grafting undernutrition and death [25,26].
In the early stage of grafting, sugars, plant hormones, small molecule metabolites, proteins, RNA, and other macromolecules can be exchanged between rootstocks and scions through the grafting interface [27,28]. The wound-healing process is affected by the interaction between rootstocks, scions, and external factors. Grafting can damage the tissues and destroy the balance of reactive oxygen species (ROS) in the plant. Then, the stress-resistant substances or genes in the rootstock may be passed upwards to the scion, improving the stress resistance of plants and rapidly repairing cell damage [29]. The antioxidant enzymes SOD and POD are important ROS scavengers [30]. This study found that the activity of SOD and POD activity increased during the early stages of grafting and the activity was the highest at 7 DAG, while SOD activity decreased to the baseline level at 14 DAG. This indicated that the wound healing process had begun and that the rootstock provided a protective enzyme to the scion, converting excess O2− generated during wound reactions. With the healing of the grafting interface, the ROS rebalanced and their activity gradually decreased. Many studies have shown that POD plays an important role in the formation of the xylem and phloem, and the activity of POD increases during grafting development [31,32], which was consistent with the findings of this study. Generally, MDA is used to assess the extent of oxidative damage and cell membrane damage. Many plant cells died at the grafted wound site, and the cells adjacent to the wound gradually degraded and necrotized to form an isolation layer, so the MDA content rapidly increased within 3 DAG. However, as the wound healed and the cell membrane regenerated, the MDA content began to decrease, returning to normal at 14 DAG. The change in protective enzyme activity and malondialdehyde content which corroborates wound healing occurred after 14 days, at which point the cells began to rebuild at the graft interface.
This research identified that the EuEG1 gene may play an important role in the wound-healing process of Eucommia ulmoides micrografting. The expression pattern of EuEG1 in the wound healing process revealed that the expression level of EuEG1 was significantly elevated in the period in which callus proliferated and decreased during the wound healing process. Additionally, EuEG1 gene overexpression can promote wound healing and improve the survival rate of grafting.
Acknowledgement: Not applicable.
Funding Statement: This study was funded by National Natural Science Foundation of China, Grant Number 31870285; Talent Special Project of Guizhou Academy of Agricultural Sciences, Grant Number 2022-02; and Talent Base for Germplasm Resources Utilization and Innovation of Characteristic Plant in Guizhou, Grant Number RCJD2018–14.
Author Contributions: Li Yi originally came up with the idea. Zhao Degang, Zhao Yichen conceived and planned the experiments. Wang Lei analyzed all data and wrote the draft manuscript. Wang Lei and Wang Runying performed experiments. All authors edited and approved the manuscript.
Availability of Data and Materials: Data supporting the reported results will be available upon request.
Ethics Approval: Not applicable.
Conflicts of Interest: The authors declare that they have no conflicts of interest to report regarding the present study.
References
1. Wu, J., Chen, H., Li, H., Tang, Y., Yang, L. (2016). Antidepressant potential of chlorogenic acid-enriched extract from Eucommia ulmoides Oliver bark with neuron protection and promotion of serotonin release through enhancing synapsin I expression. Molecules, 21(3), 260. https://doi.org/10.3390/molecules210 [Google Scholar] [CrossRef]
2. Li, Y., Wei, H., Du, K., Li, J., Zhang, Y. et al. (2020). High-quality de novo assembly of the Eucommia ulmoides haploid genome provides new insights into evolution and rubber biosynthesis. Horticulture Research, 7, 183. https://doi.org/10.1038/s41438-020-00406-w [Google Scholar] [PubMed] [CrossRef]
3. Wang, W., Zhang, X. (2017). Identification of the sex-biased gene expression and putative sex-associated genes in Eucommia ulmoides oliver using comparative transcriptome analyses. Molecules, 22(12), 22–55. https://doi.org/10.3390/molecules22122255 [Google Scholar] [PubMed] [CrossRef]
4. Li, X., Jiang, Z., Shen, Y., Li, F., Yu, X. et al. (2018). In vitro regeneration and Agrobacterium tumefaciens-mediated genetic transformation of D. lotus (Diospyros lotus L.). Scientia Horticulturae, 236, 229–237. https://doi.org/10.1016/j.scienta.2018.03.054 [Google Scholar] [CrossRef]
5. Nakazawa, Y., Toda, Y. (1995). Eucommia ulmoides Oliv. (EucommiaceaeIn vitro culture and the production of iridoids, lignans, and other secondary metabolites. In: Bajaj, Y. P. S. (ed.Medicinal and aromatic plants VIII. Biotechnology in agriculture and forestry, vol. 33. Berlin Heidelberg: Springer. https://doi.org/10.1007/978-3-662-08612-4_11 [Google Scholar] [CrossRef]
6. Glinski, M. (2006). The role of mass spectrometry in plant systems biology. Mass Spectrometry Reviews, 25, 173–214. https://doi.org/10.1002/mas.20063 [Google Scholar] [PubMed] [CrossRef]
7. Thomas, H. R., Frank, M. H. (2019). Connecting the pieces: Uncovering the molecular basis forlong-distance communication through plant grafting. New Phytologist, 223(2), 582–589. https://doi.org/10.1111/nph.15772 [Google Scholar] [PubMed] [CrossRef]
8. Robert, J., Hugard, J., Macheix, J., Martinez, J., Luis, M. C. et al. (1983). In vitro micrografting and its applications to fruit science. Scientia Horticulturae, 20(2), 147–159. https://doi.org/10.1016/0304-4238(83)90135-8 [Google Scholar] [CrossRef]
9. Navarro, L., Roistacher, C., Murashige, T. (1975). Improvement of shoot-tip grafting in vitro for virus-free citrus. Journal of the American Society for Horticultural Science, 100, 471–479. https://doi.org/10.21273/JASHS.100.5.471 [Google Scholar] [CrossRef]
10. Lane, W. D., Bhagwat, B., Armstrong, D. J., Wahlgren, S. (2003). Apple micrografting protocol to establish transgenic clones on field ready rootstock. HortTechnology Horttech, 13(4), 641–646. https://doi.org/10.1023/A:1026137224558 [Google Scholar] [CrossRef]
11. Koufan, M., Mazri, M. A., Essatte, A., Moussafir, S., Belkoura, L. et al. (2020). A novel regeneration system through micrografting for Argania spinosa (L.) skeels, and confirmation of successful rootstock-scion union by histological analysis. Plant Cell, Tissue and Organ, 142(2), 369–378. https://doi.org/10.1007/s11240-020-01868-5 [Google Scholar] [CrossRef]
12. Chilukamarri, L., Ashrafzadeh, S., Leung, D. W. M. (2021). In vitro grafting: Current applications and future prospects. Scientia Horticulturae, 280(5), 109899. https://doi.org/10.1016/j.scienta.2021.109899 [Google Scholar] [CrossRef]
13. Ashrafzadeh, S. (2020). In vitro grafting–twenty-first century’s technique for fruit tree propagation. Acta Agriculturae Scandinavica Section B–Soil and Plant Science, 70(5), 404–405. https://doi.org/10.1080/09064710.2020.1754452 [Google Scholar] [CrossRef]
14. Assunção, M., Santos, C., Brazão, J., Eiras-Dias, J. E., Fevereiro, P. (2019). Understanding the molecular mechanisms underlying graft success in grapevine. BMC Plant Biology, 19(1), 396. https://doi.org/10.1186/s12870-019-1967-8 [Google Scholar] [PubMed] [CrossRef]
15. Yıldırım, H., Onay, A., Suzerer, V., Tilkat, E., Ozden-Tokatli, Y. et al. (2010). Micrografting of almond (Prunus dulcis Mill.) cultivars “Ferragnes” and “Ferraduel”. Scientia Horticulturae, 125, 361–367. https://doi.org/10.1016/j.scienta.2010.04.022 [Google Scholar] [CrossRef]
16. Goodridge, H. S., Wolf, A. J., Underhill, D. M. (2010). β-glucan recognition by the innate immune system. Immunological Reviews, 230(1), 38–50. https://doi.org/10.1111/j.1600-065X.2009.00793.x [Google Scholar] [PubMed] [CrossRef]
17. Notaguchi, M., Kurotani, K., Sato, Y., Tabataet, R., Kawakatsu, Y. et al. (2020). Cell-cell adhesion in plant grafting is facilitated by β-1,4-glucanases. Science, 369(6504), 698–702. https://doi.org/10.1101/2020.03.26.010744 [Google Scholar] [CrossRef]
18. Kurotani, K., Chaokun, H., Koji, O., Takamasa, S., Yasunori, I. et al. (2022). Discovery of the interfamily grafting capacity of petunia, a floricultural species. Horticulture Research, 9, uhab056. https://doi.org/10.1093/hr/uhab056 [Google Scholar] [PubMed] [CrossRef]
19. Kurotani, K. I., Wakatake, T., Ichihashi, Y., Okayasu, K., Sawaiet, Y. et al. (2022). Host-parasite tissue adhesion by a secreted type of β-1,4-glucanase in the parasitic plant Phtheirospermum japonicum. Communications Biology, 3, 407. https://doi.org/10.1038/s42003-020-01143-5 [Google Scholar] [PubMed] [CrossRef]
20. Dong, X., Zhao, Y., Ran, X., Guo, L., Zhao, D. (2017). Overexpression of a new chitinase gene EuCHIT2 enhances resistance to Erysiphe cichoracearum DC. in tobacco plants. International Journal of Molecular Sciences, 18(11), 2361. https://doi.org/10.3390/ijms18112361 [Google Scholar] [PubMed] [CrossRef]
21. Fan, J., Yang, R., Li, X. (2015). The processes of graft union formation in tomato. Horticulture, Environment, and Biotechnology, 56, 569–574. https://doi.org/10.1007/s13580-015-0009-1 [Google Scholar] [CrossRef]
22. Dogra, K., Kour, K., Kumar, R., Kumar, R., Bakshi, P. et al. (2018). Graft-incompatibility in horticultural crops. International Journal of Current Microbiology and Applied Sciences, 7(2), 1805–1820. https://doi.org/10.20546/ijcmas.2018.702.218 [Google Scholar] [CrossRef]
23. Luo, G., Huang, X., Chen, J., Luo, J., Liu, Y. et al. (2022). Systematic analysis of the grafting-related glucanase-encoding GH9 family genes in pepper, tomato and tobacco. Plants, 11, 2092. https://doi.org/10.3390/plants11162092 [Google Scholar] [PubMed] [CrossRef]
24. Zhai, L., Wang, X., Tang, D., Qi, Q., Yer, H. et al. (2021). Molecular and physiological characterization of the effects of auxin-enriched rootstock on grafting. Horticulture Research, 8, 74–86. https://doi.org/10.1038/s41438-021-00509-y [Google Scholar] [PubMed] [CrossRef]
25. Milien, M., Renault-Spilmont, A. S., Cookson, S. J., Sarrazin, A., Verdeil, J. (2012). Visualization of the 3D structure of the graft union of grapevine using X-ray tomography. Scientia Horticulturae, 144, 130–140. https://doi.org/10.1016/j.scienta.2012.06.045 [Google Scholar] [CrossRef]
26. Pina, A., Errea, P., Marten, H. (2012). Graft union formation and cell-to-cell communication via plasmodesmata in compatible and incompatible stem unions of Prunus spp. Scientia Horticulturae, 50, 143–144. https://doi.org/10.1016/j.scienta.2012.06.017 [Google Scholar] [CrossRef]
27. Kanehira, A., Yamada, K., Iwaya, T., Tsuwamoto, R., Kasai, A. et al. (2010). Apple phloem cells contain some mRNAs transported over long distances. Tree Genetics & Genomes, 6(5), 635–642. https://doi.org/10.1007/s11295-010-0279-9 [Google Scholar] [CrossRef]
28. Considine, M. J., Foyer, C. H. (2021). Stress effects on the reactive oxygen species-dependent regulation of plant growth and development. Journal of Experimental Botany, 72(16), 5795–5806. https://doi.org/10.1093/jxb/erab265 [Google Scholar] [PubMed] [CrossRef]
29. Li, Y., Zhao, D. (2020). Transcriptome analysis of grafted potato rootstock for improvement of scion late-blight resistance. BMC Plant Biology, 21(272), 5–13. https://doi.org/10.1186/s12870-021-03039-w [Google Scholar] [PubMed] [CrossRef]
30. Chrysargyris, A., Michailidi, E., Tzortzakis, N. (2018). Physiological and biochemical responses of Lavandula angustifolia to salinity under mineral foliar application. Frontiers in Plant Science, 9, 489. https://doi.org/10.3389/fpls.2018.00489 [Google Scholar] [PubMed] [CrossRef]
31. Martínez, B., Alcaraz, L., Muries, B., Cadenaset, M., Carvajal, M. (2010). Physiological aspects of rootstock-scion interactions. Scientia Horticulturae, 127(2), 112–118. https://doi.org/10.1016/j.scienta.2010.08.002 [Google Scholar] [CrossRef]
32. Fernández, N., Martínez, V., Carvajal, M. (2004). Effect of salinity on growth, mineral composition, and water relations of grafted tomato plants. Journal of Plant Nutrition & Soil Science, 167(5), 616–622. https://doi.org/10.1002/jpln.200420416 [Google Scholar] [CrossRef]
Cite This Article
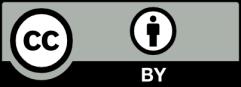