Open Access
ARTICLE
Responses of Wheat Production, Quality, and Soil Profile Properties to Biochar Applied at Different Seasons in a Rice-Wheat Rotation
Department of Horticulture, Foshan University, Foshan, 528000, China
* Corresponding Author: Hongdong Xiao. Email:
(This article belongs to the Special Issue: Enhancing the Carbon Sequestration Capacity of the Agricultural, Forestry, and Agroforestry Ecosystems)
Phyton-International Journal of Experimental Botany 2023, 92(12), 3359-3370. https://doi.org/10.32604/phyton.2023.046877
Received 17 October 2023; Accepted 14 November 2023; Issue published 28 December 2023
Abstract
In the rice-wheat rotation system, biochar (BC) can be applied at the initiation of the rice or wheat season. Here, we compared the effects of BC that were applied at two different crop seasons on wheat production, quality, and soil profile properties in a rice-wheat rotation system with nitrogen (N) fertilizer applied at 280 kg/ha rate. Results showed that both wheat grain production and N recovery use efficiency were influenced by BC applied at two crop seasons. Biochar application did not affect the total non-essential amino-acid, but when applied during wheat season, BC significantly (p < 0.05) increased total essential amino acid in grain by 12.3%, particularly for the valine (+48.2%), methionine (+43.8%), and isoleucine (+10.3%). We found that BC significantly (p < 0.05) decreased the pH of soil at 0–6 cm and 20–30 cm by 0.14–0.18 and 0.05–0.08 units, respectively. The NH4+-N content of the whole observed soil profile were reduced by BC application, however, the effect of BC on NO3–-N content varied with the application season and profile depth. Interestingly, BC applied at wheat and rice season significantly (p < 0.05) improved topsoil N contents by 48.4% and 19.7%, respectively. In addition, data suggested that BC applied during wheat season performed better in enhancing soil available phosphorus, potassium, and organic matter contents. In conclusion, we suggest that the optimum application time of BC for enhancing crop production and quality (take amino-acid content for example) and improving soil fertility is at the initiation of the wheat season.
Keywords
The use of chemical fertilizers, in particular nitrogen (N), is a key to food security all over the world [1], where grain production increased by 74% between 1982 and 2020 [2]. However, with the dramatically increasing use of N fertilizer, Chinese policymakers and landowners recognize the interconnections between water and air environmental pollution, low N use efficiency (NUE), and degraded soil fertility [3,4]. It is therefore vital to improve the NUE and soil properties for stable food crops such as maize (Zea mays), wheat (Triticum aestivum and Triticum durum), and rice (Oryza sativa) that receive approximately 45% of the applied chemical N fertilizer all over the world [3,5]. Previous works demonstrated that optimizing N fertilizer and irrigation management and amending soil additives could help to address the forgoing issues in stable crop and vegetable productions [6–10].
Biochar (BC) is a typical carbon-rich product that is derived from the thermochemical conversion of agriculture and forest biomass or others under oxygen-limited conditions [11]. Its use in agricultural systems comes with numerous benefits such as improving stable crop yields, ameliorating key soil properties, and combating global climate change [11,12]. Agronomic BC research is a rapidly evolving research field, with >15,000 publications at the end of 2020 but with only <100 publications in 2010 [13,14]. There is no doubt that BC will be broadly used in future agronomic practices. In field management of farmland owners, BC is always incorporated with the topsoil or plow layer while conducting tillage. In China, rice-wheat, maize-wheat, and rice-rape are three typical crop rotations [3,15]. To improve crop production and increase the net benefits of farmland systems, landowners always practice tillage management at one of the crop seasons of rotation. In the rice-wheat rotation system, it means that BC might be incorporated into the paddy soil at the initiation of the rice or wheat growth cycle. Under this context, the alternations of aerobic and anaerobic environments as a result of overlying water flooded and drained change the properties of BC that are applied before either rice transplanting or wheat sowing. These changes in the properties of aged BC affect its functions on crop production and soil characteristics [16,17]. Nevertheless, studies comparing the effects of BC applied at different stages of the crop growth cycle of a rice-wheat rotation system are lacking.
Although the changes in characteristics of soil from BC-amended soil had been well documented [18], understanding the changes in soil fertility caused by BC application at soil profile is limited. Factors influencing soil fertility including available nutrients, pH, and soil organic matter (SOM) can vary dramatically along with profile depth [19,20]. Liu et al. evaluated the ability of BC that is derived from maize straw to mitigate the N and phosphorus (P) losses and improve the distribution of BC in soil profile [21]. In addition, Ingunn et al. carried out a NIR hyper-spectral reflectance imaging and identified the distribution pattern of BC particles along with the soil profile [22]. The vertical movement of BC particles no doubt changes some soil properties at profile.
In the light of above, we conducted a pot experiment with PVC columns to investigate how crop production, quality, and soil profile properties respond to BC that is applied at the initiations of the rice or wheat growth cycle. We hypothesized that BC applied at different crop seasons would exert varying effects on soil fertility at the profile level. Results obtained from current work would help us to comprehensively clarify how BC management affects crop production and soil fertility in the rice-wheat rotation system.
2.1 Soil Column Preparation and BC Properties
In a greenhouse with a top-shed at Yixing, China, a soil pot experiment using a PVC column (with 30 cm in inner diameter and 60 cm in height) was conducted. To keep the same conditions with the ambient environment such as the wind, temperature, and moisture, no walls were installed in the greenhouse during crop growing cycle. The study area had an average annual precipitation of 1120 mm and an annual temperature of 16.0°C. Tested soil was classified as a typical Hydragric Anthrosol, sampled from 0–20 cm topsoil and 20–50 cm subsoil in a rice-wheat rotation located at Zhoutie Town (31° 28′N, 119° 59′E), Yixing, Jiangsu Province, China. After air drying and sieved by a 2-mm nylon mesh, the 0–20 and 20–50 cm soil samples were separately repacked into the soil columns. The 0–20 cm topsoil had the following basic properties: pH 7.7 (soil:water = 1:5), bulk density 1.31 g/cm3, available N 96 mg/kg, available P 53 mg/kg, available potassium (K) 188 mg/kg, and organic matter 23.2 g/kg.
The BC used in the current study was provided by Nanjing Forestry University and derived from dried wheat straw, using a biogas-energy pyrolysis system at 500°C continuing 8 h. The physicochemical characteristics of the fresh BC before being incorporated into the soil were pH 9.1 (BC:H2O = 1:5), CEC (cation exchange capacity) 23 cmol/kg, total carbon 480 g/kg, total N 7.3 g/kg, and BET surface area 32.0 m2/g. The BC application rate was 35 g/pot, approximately equivalent to 5 t/ha under field condition. We mixed the BC and 0–20 topsoil before rice transplanting and wheat sowing in June and November 2019, respectively. The corresponding experimental treatments were labeled as R and W, respectively. The same management was done consistently during each crop season until the wheat was harvested on May 16, 2021.
2.2 Experiment Design and Management
The current pot experiment included three treatments: (1) chemical N fertilizer (urea) applied at 280 kg/ha rate (abbreviated as N280) for each rice-wheat rotation (180 and 100 kg/ha for rice and wheat, respectively); and (2, 3) urea N plus 5 t/ha BC at initiations of rice [N280+BC(R)] or wheat [N280+BC(W)] growth cycles. For all treatments, besides urea-N, calcium superphosphate, and potassium sulfate were applied into 0–20 cm topsoil to supply P and K nutrients for plant growth at rates of 120 kg/ha P2O5 and 100 kg/ha K2O, respectively. Both P and K fertilizers were applied as basal fertilizers.
2.3 Soil and Plant Sampling and Determinations
For the current study, we used a soil auger (5 cm inner diameter) to collect the 0–6, 6–12, 12–20, 20–30, 30–40, and 40–50 cm depth soil samples from 5 random and different positions in each pot of the control and BC-added treatments) on June 10, 2021, after wheat harvest. The soils from five positions were pooled together to form a composite sample. We first picked out the roots in fresh soils and then sieved them through 2 mm nylon mesh. The soil samples were stored at a 4°C refrigerator before determining their properties.
Soil pH (1:5 soil-water ratio) was determined using a pH probe (Quark Ltd., Nanjing, China). Soil total N and SOM were measured by a CN analyzer (Perkin Elmer, 2400 II, Waltham, USA) and wet digestion method with H2SO4-K2Cr2O7, respectively. We used 2 M KCl (1:5 soil-solution ratio) to extract the exchangeable NH4+-N and NO3−-N and then quantified them by a continuous-flow San++ System analyzer (Skalar Analytical BV, Breda, Netherlands).
Wheat shoots were manually harvested after plants reached their maturity (end of May 2021) and divided into grain and straw, respectively. After being oven-dried, their dry weights were recorded. Meanwhile, separately grain and straw (approx. 300 g for each) were oven dried at 105°C for 0.5 h firstly and then at 75°C to a constant weight. The total N contents of the dry plant sample were determined by the Kjeldahl method, after being digested with H2O2 and H2SO4 solutions. Wheat N uptake capacity was calculated as the multiplied product of the dry biomass weight and total N contents. The percentage of wheat biomass (straw plus grain) N accumulation increases because urea application to the N rate was used as wheat NUE [23]. The free amino-acid content determination and the total amino-acid content calculation of wheat grain were conducted as described elsewhere [23].
Data in tables and figures were presented as the means (n = 3) ± standard deviations (SDs). We determined the statistically significant differences between the treatments using the single-factor ANOVA method. The least significant difference (LSD) multiple comparison method in SPSS 16.0 software was applied at p < 0.05 level.
Wheat plant height, straw dry biomass, and grain production under three treatments were 68.7–69.3 cm, 45.0–47.9 g/pot, and 29.7–31.9 g/pot, respectively, which were not influenced by BC, regardless of the crop seasons in a rice-wheat rotation (Table 1). Increasing crop production is always the first commonly reported positive effect of amending BC into farmland soils [13]. Improvements in the physical properties of soil such as bulk density and water holding capacity might decide the effects of BC on grain yield. However, some contrasting experimental results have been published, which are dependent on their experimental scale, soil characteristics, and BC type [24]. Unlike our result, Hu et al. reported a remarkable after-effect of straw-derived BC in improving crop plant growth and final production [15]. No positive effect in wheat grain yield improvement following BC amendments may be explained by the sufficient N nutrient in tested soil [5,25]. What was more, our data showed that applying BC during the wheat season, i.e., the N280+BC(W) treatment, significantly (p < 0.05) decreased the thousand-seed weight of wheat grain by 11.6%, compared with N280. However, three yield-related agronomic traits of wheat grain were not changed by BC and were amended before rice seedlings were transplanted.
Wheat NUE ranged from 52.2% to 59.7% (Table 1). Wheat planted under N280+BC(R) had the highest NUE, which was 12.6% higher than that under N280+BC(W). However, no difference in wheat NUE among the three treatments was statistically significant (p < 0.05). According to a previous meta-analysis, BC leads to an average increase in plant N uptake by 11%, which is attributed to a remarkable 12% increase in plant biomass but a minor 2% decrease in plant tissue N content [26].
3.2 Free Amino Acid Content in Wheat Grain
Biochar addition increased the total free amino acid contents in wheat grain (Fig. 1). Compared with N280, N280+BC(W) significantly (p < 0.05) improved (+4.0%) the content of free total amino acid in wheat grain. The balance of free amino acid compositions contained in the edible wheat grains determines their nutritional quality and market price [27]. Similar to a study conducted in saline soil [23], the main components of the total amino acids were influenced by BC amendment. Easily understood, the ambient environmental conditions influence crop production, protein quantity, and amino acid compositions [28]. Meanwhile, improvements in soil physical properties such as the bulk density and water holding capacity following the BC amendment might promote the amino acid content of wheat grain. Therefore, BC applied at an optimum time can improve crop grain quality. Additional studies are required to assess the long-term effects of BC application on the amino acid content of wheat and other edible quality, particularly under field conditions.
Figure 1: Response of total amino-acid content of wheat to biochar (BC) applied at rice (R) or wheat (W) season. Data were presented as means ± SD (n = 3); data labeled with different small letters is significantly different at p < 0.05 level
Crop grain amino acids can be classified into two major kinds: essential amino acids and non-essential amino acids, which vary from 8%–26% and depend on the crop genotype and the field growing conditions [29]. Data from Table 2 showed that the BC being applied before wheat seed sowing significantly (p < 0.05) increased the contents of valine (+48.2%), methionine (+43.8%), and isoleucine (+10.3%), compared with N280. In addition, 26.1% more lysine was recorded from N280+BC(R) than from N280 (significant at p < 0.05). Other essential amino acids in wheat grain, i.e., threonine, leucine, and phenylalanine were not impacted by BC applied at either crop season. Overall, BC amendment improved the essential amino-acid content in wheat grain by 0.11–0.26 mg/kg. Particularly, wheat grain harvested from N280+BC(W) had 11.5% more essential amino acid than that from N280 (significant at p < 0.05; Table 2). Therefore, from the perspective of improving grain quality, the BC applied during the wheat growth cycle exerted a greater positive effect.
By contrast, BC applied at both crop seasons exerted a weak effect on the contents of non-essential amino acids in wheat grain (Table 3). Only glycine was decreased significantly (p < 0.05) by 17.2% following BC that was added during the wheat season.
For N280, the 0–6 cm topsoil layer had a pH of 7.26, then it decreased to 7.08–7.12 in the subsoil profile. Data from Fig. 2 demonstrated that the BC applied at both crop seasons significantly (p < 0.05) decreased the 0–6 and 20–30 cm soil pH by 0.14–0.18 and 0.05–0.08 units, respectively. At 6–12 cm, the BC applied at two varied crop seasons exerted a contrasting influence on soil pH. Only being applied at the rice season, BC reduced the 30–40 cm soil pH by 0.07 units. Furthermore, the pH of soil collected from the 12–20 and 40–50 cm was not influenced by BC, regardless it was added during rice or wheat season. Generally, previous studies have confirmed that BC amending treatments showed remarkable increases in soil pH as compared with the control [30,31]. Reduced pH soil that mixed with BC is the result of its basic characteristic. However, along with the aging process in soil, the pH of BC decreased by 1.6–3.8 units, according to Bakshi et al. [32], which helps to explain the relative lower pH in BC-amended soils in the current work.
Figure 2: Changes in pH of soil profile caused by biochar (BC) applied at different crop seasons. Data were presented as means ± SDs (n = 3); data labeled with different small letters for each depth is significantly different at p < 0.05 level
3.3.2 NH4+-N, NO3−-N and Total N
Generally, the soil NH4+-N contents were 0.33–0.88 mg/kg at the 0–6 cm topsoil, which increased to 1.70–2.20 mg/kg at the 6–12 cm profile. Data in Fig. 3 suggested that the BC application overall lowered the soil NH4+-N contents. In particular, the NH4+-N contained in the 0–6, 30–40, and 40–50 cm soil from N280+BC(R) and N280+BC(W) were 47.7%–62.5%, 61.1%–77.8%, and 27.8%–40.7% lower than that from N280 (significant at p < 0.05). Furthermore, BC applied during rice season reduced the NH4+-N content (−22.7%) of the 6–10 cm soil profile. It is a critical property of BC’s sorption on nutrients such as NH4+-N, which helps to explain its positive value as a soil additive [17]. According to Liao et al. [33], aged BC had a significantly higher NH4+ immobilization rate than fresh BC (over 4-folds), which process was primarily dominated by immobilizing NH4+ into the recalcitrant organic N pool. This phenomenon helps us to understand the relative lower NH4+ content in topsoil as a result of BC amending.
Figure 3: Changes in ammonium (NH4+-N), nitrate (NO3–-N), and total N (TN) contents of soil profile caused by biochar (BC) applied at rice (R) or wheat (W) crop season. Data were presented as means ± SD (n = 3); different small letters in the same profile refer to significant differences between treatments at p < 0.05 level
The influence of BC application on soil NO3–-N content was relatively weak (Fig. 3). Only at 20–30 and 40–50 cm soil profiles, N280+BC(W) and N280+ BC(R) had 46.9% and 76.9% more NO3−-N content than N280, respectively (both significant at p < 0.05). Biochar has shown the potential to reduce N-leaching losses from fertilizer sources in farmland soil [34]. Interestingly, our data suggested that BC amendment generally increased soil total N content in the profile. Particularly, BC amendments enhanced total N content from the 0–6 and 40–50 cm soils by 19.7%–48.4% and 36.6%–57.7%, respectively (Fig. 3). Plants in N280+BC(W) treatment also had 24.0% and 7.3% higher total N than N280+BC(R) at the 0–6 and 20–30 cm soil depth, respectively (significant at p < 0.05). This result indicated that wheat season may be the better time to apply BC into paddy soil for N retention. Similarly, Han et al. clarified the optimum BC application schemes in a maize-wheat rotation considering crop production efficiency and soil fertility [35].
3.3.3 Available P and K Contents in Soil
At the 0–6 cm topsoil, BC amendment lowered soil available P content, particularly under N280+BC(R) treatment, which with 16.8% lower available P content than N280 (Fig. 4). Nevertheless, BC applied at rice season increased soil available P content at the 6–12 cm horizon. At other soil profiles, BC additives overall increased the available P contents. The BC applied before wheat seed sowing increased the available P contents in the 12–20, 20–30, and 40–50 cm soils by 30.4%, 41.5%, and 30.1%, respectively (all significant at p < 0.05). Similarly, soil available K contents were enhanced by the BC, particularly that being applied during the wheat season. For instance, the available K contents in N280+BC(W) soils from 0–6, 6–12, and 30–40 cm were 44.2%, 46.3%, and 22.0% higher than that in N280 (Fig. 4). However, two BC amending treatments also significantly (p < 0.05) decreased the available K contents of 40–50 cm (on average by 26.4%).
Figure 4: Changes in available P (AP) and K (AK) contents of soil profile caused by biochar (BC) applied at rice (R) or wheat (W) season. Data were presented as means ± SDs (n = 3); different small letters in the same profile refer to significant differences between treatments at p < 0.05 level
Similarly, the BC additive remarkably raised the available P content in the soil, in comparison with the control condition from a previous indoor incubation experiment [36]. However, the soil available P concentration varied little in BC-amended treatment but decreased in straw-amended treatment according to a 7-year long-term investigation [37]. These results demonstrated that BC amendment is favorable for available P nutrients in the soil, at least compared with straw turnover. Biochar also has been approved to substitute partially P and K fertilizers with appropriate rates in the farming systems [38], which also indicated that BC amendments could enhance soil fertility. Moreover, our results suggested that the positive benefit in improving soil available P and K nutrients of BC applied during the wheat season is better than that applied during the rice season (Fig. 4). Of course, more long-term field experiments should be conducted in the future.
There was an average of 108.7% and 46.1% more SOM contained in the 0–6 and 6–12 cm layers under BC-added treatments, respectively, compared with that under N280 (Fig. 5). Overall, N280+BC(W) had more SOM than N280+BC(R), in particular, that was recorded at the 0–6 cm topsoil and 20–30 cm subsoil. Biochar addition increases soil organic carbon content [38]. In addition, a seven-year continuous BC amendment could favor the soil organic carbon by increasing the aromatic [37]. We demonstrated the SOM-increasing effects following the BC amendment could be observed to cover a 0–50 cm soil profile. Similarly, previous work confirmed the potential of BC to improve SOM along with the whole soil profile [39]. Biochar has been widely produced and applied to sequestrate carbon and remediate degraded soil as described in the introduction section. The increase in SOM because of BC can be explained by the BC-contained carbon itself and it could inhibit the SOM mineralization while promoting the root volume [40,41].
Figure 5: Changes in soil organic matter (SOM) content at different depths caused by biochar (BC) applied at rice (R) or wheat (W) crop season. Data were presented as means ± SDs (n = 3); different small letters in the same profile refer to significant differences between treatments at p < 0.05 level
We conducted a simulated soil pot experiment using PVC columns to clarify how BC that applied at the initiation of the rice or wheat growth cycle on wheat production, quality (amino acid content), and soil properties at 0–50 cm depth in a rice-wheat rotation. Biochar did not influence the wheat grain yield and recovery NUE, but when applied during the wheat season, BC enhanced some essential amino acids in wheat grain, which helped to improve the grain quality. The changes in the soil characteristics caused by BC were mainly recorded at the 0–12 cm topsoil, and the effects were dependent on the applying time of BC. In particular, the total N and SOM contained in the 0–6 cm topsoil were enhanced by BC application at both crop seasons, which effect was greater in treatment with BC that is applied during wheat season. Biochar amendments still changed the characteristics of the 12–50 cm subsoil, but the effects were weaker than that on the topsoil. Overall, we conclude that it is better to apply BC during the wheat season to improve crop quality and optimize soil fertility along the soil profile. Meanwhile, long-term and field condition studies should be conducted.
Acknowledgement: We thank Sergey Shabala (University of Western Australia) for improving the English language of the manuscript.
Funding Statement: This work was financially supported by the Higher Education Department of Guangdong Province (No. 2020KCXTD025) and the National Key Research and Development Program of China (No. 2017YFD0800404).
Author Contributions: Study conception and design: H.X.; data collection: L.C., R.D.; analysis and interpretation of results: L.C., X.L., and H.X.; draft manuscript preparation and review: L.C., M.Y., and H.X. All authors reviewed the results and approved the final version of the manuscript.
Availability of Data and Materials: All data included in this work are available upon request by contact with the corresponding author.
Ethics Approval: Not applicable.
Conflicts of Interest: The authors declare that they have no conflicts of interest to report regarding the present study.
References
1. Yi, Z., Jeyakumar, P., Jiang, J., Zhang, X., Yue, C. et al. (2022). Effect of reaction-finished solution of hydrochar (HRFS) application on rice grain yield and nitrogen use efficiency in saline soil. Phyton-International Journal of Experimental Botany, 91(4), 859–868. https://doi.org/10.32604/phyton.2022.018396 [Google Scholar] [CrossRef]
2. Guo, Y., Chen, Y., Searchinger, T. D., Zhou, M., Pan, D. et al. (2020). Air quality, nitrogen use efficiency, and food security in China are improved by cost-effective agricultural nitrogen management. Nature Food, 1(10), 648–658. https://doi.org/10.1038/s43016-020-00162-z [Google Scholar] [PubMed] [CrossRef]
3. Yu, X., Keitel, C., Zhang, Y., Wangeci, A. N., Dijkstra, F. A. (2022). Global meta-analysis of nitrogen fertilizer use efficiency in rice, wheat and maize. Agriculture, Ecosystems and Environment, 338, 108089. https://doi.org/10.1016/j.agee.2022.108089 [Google Scholar] [CrossRef]
4. Zheng, X., Liu, Q., Cao, M., Ji, X., Lu, J. et al. (2022). Nitrogen uptake by plants may alleviate N deposition-induced increase in soil N2O emissions in subtropical Chinese fir (Cunninghamia lanceolata) plantations. Plant and Soil, 479(1–2), 127–142. https://doi.org/10.1007/s11104-022-05503-4 [Google Scholar] [CrossRef]
5. Wu, S., Zhang, Z., Sun, H., Hu, H. (2023). Responses of rice yield, N uptake, NH3 and N2O losses from reclaimed saline soils to varied N inputs. Plants, 12(13), 2446. https://doi.org/10.3390/plants12132446 [Google Scholar] [PubMed] [CrossRef]
6. Zhang, X., Gu, B., van Grinsven, H., Lam, S. K., Liang, X. et al. (2020). Societal benefits of halving agricultural ammonia emissions in China far exceed the abatement costs. Nature Communications, 11(1), 4357. https://doi.org/10.1038/s41467-020-18196-z [Google Scholar] [PubMed] [CrossRef]
7. Chen, S., Li, D., He, H., Zhang, Q., Lu, H. et al. (2022). Substituting urea with biogas slurry and hydrothermal carbonization aqueous product could decrease NH3 volatilization and increase soil DOM in wheat growth cycle. Environmental Research, 214(2), 113997. https://doi.org/10.1016/j.envres.2022.113997 [Google Scholar] [PubMed] [CrossRef]
8. Xu, J., Min, J., Sun, H., Singh, B. P., Wang, H. et al. (2022). Biostimulants decreased nitrogen leaching and NH3 volatilization but increased N2O emission from plastic-shed greenhouse soil. Environmental Science and Pollution Research, 29(4), 6093–6102. https://doi.org/10.1007/s11356-021-16039-y [Google Scholar] [PubMed] [CrossRef]
9. Yi, Z., Jeyakumar, P., Yin, C., Sun, H. (2023). Effects of biochar in combination with N inputs on grain yield, N uptake, NH3 volatilization, and N2O emission in paddy soil. Frontiers in Microbiology, 14, 1174805. https://doi.org/10.3389/fmicb.2023.1174805 [Google Scholar] [PubMed] [CrossRef]
10. Chen, Y., Zhang, S., Du, S., Zhang, X., Wang, G. et al. (2022). Effects of exogenous potassium (K+) application on the antioxidant enzymes activities in leaves of Tamarix ramosissima under NaCl stress. Genes, 13(9), 1507. https://doi.org/10.3390/genes13091507 [Google Scholar] [PubMed] [CrossRef]
11. Wu, P., Ata-Ul-Karim, S. T., Singh, B. P., Wang, H., Wu, T. et al. (2019a). A scientometric review of biochar research in the past 20 years (1998–2018). Biochar, 1(1), 23–43. https://doi.org/10.1007/s42773-019-00002-9 [Google Scholar] [CrossRef]
12. Lehmann, J., Cowie, A., Masiello, C. A., Kammann, C., Woolf, D. et al. (2021). Biochar in climate change mitigation. Nature Geoscience, 14(12), 883–892. https://doi.org/10.1038/s41561-021-00852-8 [Google Scholar] [CrossRef]
13. Schmidt, H., Kammann, C., Hagemann, N., Leifeld, J., Bucheli, T. D. et al. (2021). Biochar in agriculture—A systematic review of 26 global meta-analyses. Global Change Biology Bioenergy, 13(11), 1708–1730. https://doi.org/10.1111/gcbb.12889 [Google Scholar] [CrossRef]
14. Zhang, J., Zhang, S., Niu, C., Jiang, J., Sun, H. (2022). Positive effects of biochar on the degraded forest soil and tree growth in China: A systematic review. Phyton-International Journal of Experimental Botany, 91(8), 1601–1616. https://doi.org/10.32604/phyton.2022.020323 [Google Scholar] [CrossRef]
15. Hu, Y., Sun, B., Wu, S., Feng, H., Gao, M. et al. (2021). After-effects of straw and straw-derived biochar application on crop growth, yield, and soil properties in wheat (Triticum aestivum L.)-maize (Zea mays L.) rotations: A four-year field experiment. Science of the Total Environment, 780(10), 146560. https://doi.org/10.1016/j.scitotenv.2021.146560 [Google Scholar] [PubMed] [CrossRef]
16. Zhang, M., Riaz, M., Zhang, L., El-desouki, Z., Jiang, C. (2019). Biochar induced changes to basic soil properties and bacterial communities of different soils to varying degrees at 25 mm rainfall: More effective on acidic soils. Front Microbiol, 10, 1321. https://doi.org/10.3389/fmicb.2019.01321 [Google Scholar] [PubMed] [CrossRef]
17. Weldon, S., van der Veen, B., Farkas, E., Kocatürk-Schumacher, N. P., Dieguez-Alonso, A. et al. (2022). A re-analysis of NH4+ sorption on biochar: Have expectations been too high? Chemosphere, 301(2), 134662. https://doi.org/10.1016/j.chemosphere.2022.134662 [Google Scholar] [PubMed] [CrossRef]
18. Lu, T., Wang, X., Du, Z., Wu, L. (2021). Impacts of continuous biochar application on major carbon fractions in soil profile of North China Plain’s cropland: In comparison with straw incorporation. Agricultural, Ecosystems & Environment, 315, 107445. https://doi.org/10.1016/j.agee.2021.107445 [Google Scholar] [CrossRef]
19. Elmi, A. A., Madramootoo, C., Hamel, C., Liu, A. (2003). Denitrification and nitrous oxide to nitrous oxide plus dinitrogen rations in the soil profile under three tillage systems. Biology and Fertility of Soils, 38(6), 340–348. https://doi.org/10.1007/s00374-003-0663-9 [Google Scholar] [CrossRef]
20. Hansel, C. M., Fendorf, S., Jardine, P. M., Francis, C. A. (2008). Changes in bacterial and archaeal community structure and functional diversity along a geochemically variable soil profile. Applied and Environmental Microbiology, 74(5), 1620–1633. https://doi.org/10.1128/AEM.01787-07 [Google Scholar] [PubMed] [CrossRef]
21. Liu, S., Cao, D., Hang, Y., Ge, S., He, W. et al. (2022). Biochar affects the distribution of phosphorus in the soil profile by changing the capacity of soil to adsorb phosphorus and its distribution within soil macroaggregates. Land Degradation & Development, 34(8), 2189–2200. https://doi.org/10.1002/ldr.4599 [Google Scholar] [CrossRef]
22. Ingunn, B., Christophe, M., Andreas, F., Cecilia, F., Markus, S. et al. (2016). Qualitative and quantitative mapping of biochar in a soil profile using hyperspectral imaging. Soil & Tillage Research, 155(2), 523–531. https://doi.org/10.1016/j.still.2015.06.020 [Google Scholar] [CrossRef]
23. Sun, H., Zhang, H., Shi, W., Zhou, M., Ma, X. (2019). Effect of biochar on nitrogen use efficiency, grain yield and amino acid content of wheat cultivated on saline soil. Plant, Soil and Environment, 65(2), 83–89. https://doi.org/10.17221/525/2018-PSE [Google Scholar] [CrossRef]
24. Jeffery, S., Verheijen, F. G. A., van der Velde, M., Bastos, A. C. (2011). A quantitative review of the effects of biochar application to soils on crop productivity using meta-analysis. Agriculture, Ecosystem and Environment, 144(1), 175–187. https://doi.org/10.1016/j.agee.2011.08.015 [Google Scholar] [CrossRef]
25. Xie, Y., Dong, C., Chen, Z., Liu, Y., Zhang, Y. et al. (2020). Successive biochar amendment affected crop yield by regulating soil nitrogen functional microbes in wheat-maize rotation farmland. Environmental Research, 194, 110671. https://doi.org/10.1016/j.envres.2020.110671 [Google Scholar] [PubMed] [CrossRef]
26. Liu, Q., Zhang, Y., Liu, B., Amonette, J. E., Lin, Z. et al. (2018). How does biochar influence soil N cycle? A meta-analysis. Plant and Soil, 426(1–2), 211–225. https://doi.org/10.1007/s11104-018-3619-4 [Google Scholar] [CrossRef]
27. Zhang, M., Ma, D., Wang, C., Zhao, H., Zhu, Y. et al. (2016). Responses of amino acid composition to nitrogen application in high- and low-protein wheat cultivars at two planting environments. Crop Science, 56(3), 1277–1287. https://doi.org/10.2135/cropsci2015.08.0504 [Google Scholar] [CrossRef]
28. Kovács, Z., Simon-Sarkadi, L., Sovány, C., Kirsch, K., Galiba, G. et al. (2011). Different effects of cold acclimation and abscisic acid on free amino acid composition in wheat. Plant Science, 180(1), 61–68. https://doi.org/10.1016/j.plantsci.2010.08.010 [Google Scholar] [PubMed] [CrossRef]
29. Gafurova, D. A., Tursunkhodzhaev, P. M., Kasymova, T. D., Yuldashev, P. K. (2002). Fractional and amino-acid composition of wheat grain cultivated in Uzbekistan. Chemistry of Natural Compounds, 38(5), 462–465. https://doi.org/10.1023/A:1022167811596 [Google Scholar] [CrossRef]
30. Liu, J., Shen, J., Li, Y., Su, Y., Ge, T. et al. (2014). Effects of biochar amendment on the net greenhouse gas emission and greenhouse gas intensity in a Chinese double rice cropping system. European Journal of Soil Biology, 65(56), 30–39. https://doi.org/10.1016/j.ejsobi.2014.09.001 [Google Scholar] [CrossRef]
31. Wu, Z., Zhang, X., Dong, Y., Li, B., Xiong, Z. (2019b). Biochar amendment reduced greenhouse gas intensities in the rice-wheat rotation system: Six-year field observation and meta-analysis. Agricultural and Forest Meteorology, 278(1), 107625. https://doi.org/10.1016/j.agrformet.2019.107625 [Google Scholar] [CrossRef]
32. Bakshi, S., Aller, D. M., Laird, D. A., Chintala, R. (2016). Comparison of the physical and chemical properties of laboratory and field-aged biochars. Journal of Environmental Quality, 45(5), 1627–1634. https://doi.org/10.2134/jeq2016.02.0062 [Google Scholar] [PubMed] [CrossRef]
33. Liao, X., Müller, C., Jansen-Willems, A., Luo, J., Lindsey, S. et al. (2021). Field-aged biochar decreased N2O emissions by reducing autotrophic nitrification in a sandy loam soil. Biology and Fertility of Soils, 57(4), 471–483. https://doi.org/10.1007/s00374-021-01542-8 [Google Scholar] [CrossRef]
34. Sika, M. P., Hardie, A. G. (2013). Effect of pine wood biochar on ammonium nitrate leaching and availability in a South African sandy soil. European Journal of Soil Science, 65(1), 113–119. https://doi.org/10.1111/ejss.12082 [Google Scholar] [CrossRef]
35. Han, X., Wang, X., Hou, K., Jia, F., Sun, Y. et al. (2019). Application period and dosage optimums for biochar addition in black soil with summer maize. Journal of Zhejiang A&F University, 36(1), 96–106 (In Chinese). https://doi.org/10.11833/j.issn.2095-0756.2019.01.013 [Google Scholar] [CrossRef]
36. Safian, M., Motaghian, H., Hosseinpur, A. (2021). Effect of sugarcane residue biochar and P fertilizer on P availability and its fractions in a calcareous clay loam soil. Biochar, 2(3), 357–367. https://doi.org/10.1007/s42773-020-00050-6 [Google Scholar] [CrossRef]
37. Yuan, J., Wang, Y., Zhao, X., Chen, H., Chen, G. et al. (2021). Seven years of biochar amendment has a negligible effect on soil available P and a progressive effect on organic C in paddy soils. Biochar, 4(1), 1. https://doi.org/10.1007/s42773-021-00127-w [Google Scholar] [CrossRef]
38. Nobile, C., Lebrun, M., Védère, C., Honvault, N., Aubertin, M. et al. (2022). Biochar and compost addition increases soil organic carbon content and substitutes P and K fertilizer in three French cropping systems. Agronomy for Sustainable Development, 42(6), 119. https://doi.org/10.1007/s13593-022-00848-7 [Google Scholar] [CrossRef]
39. Cao, D., Lan, Y., Sun, Q., Yang, X., Chen, W. et al. (2021). Maize straw and its biochar affect phosphorus distribution in soil aggregates and are beneficial for improving phosphorus availability along the soil profile. European Journal of Soil Science, 72(5), 2165–2179. https://doi.org/10.1111/ejss.13095 [Google Scholar] [CrossRef]
40. Cheng, H., Hill, P. W., Bastami, M. S., Jones, D. L. (2017). Biochar stimulates the decomposition of simple organic matter and suppresses the decomposition of complex organic matter in a sandy loam soil. GCB Bioenergy, 9(6), 1110–1121. https://doi.org/10.1111/gcbb.12402 [Google Scholar] [CrossRef]
41. Li, F., Zhou, J., Guo, X., Feng, S., Wang, L. et al. (2023). Effect of biochar and earthworms on mineralization of organic matter in top soil and deep soil. Environmental Engineering Science, 40(8), 340–346. https://doi.org/10.1089/ees.2023.0069 [Google Scholar] [CrossRef]
Cite This Article
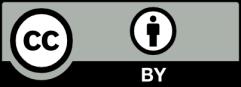