Open Access
REVIEW
Application of Silicon and Selenium in Rice for Reducing Cadmium Stress
College of Environment and Surveying and Mapping Engineering, Suzhou University, Suzhou, 234000, China
* Corresponding Author: Haiyang Zhang. Email:
(This article belongs to the Special Issue: Identification of Genetic/Epigenetic Components Responding to Biotic and Abiotic Stresses in Crops)
Phyton-International Journal of Experimental Botany 2023, 92(6), 1873-1886. https://doi.org/10.32604/phyton.2023.027924
Received 25 November 2022; Accepted 09 February 2023; Issue published 11 April 2023
Abstract
Rice is an essential part of the human diet in most parts of the world; On the other hand, the industrialization of societies has led to pollution of the environment, including heavy metal contamination of soil and water, which negatively affects rice production and quality. Therefore, finding ways to increase the yield and quality of this strategic crop seems essential. Several studies have been conducted in recent decades to find effective and inexpensive solutions to reduce the adverse effects of heavy metals in rice fields. Due to the negative effect of cadmium pollution on rice quality and yield, the current study aimed to investigate cadmium absorption and transfer mechanisms in rice (from absorption by roots to loading in grains), and its effects on rice morphology, physiology, and biochemistry (such as biomass, nutrient absorption, antioxidant defenses). Also, rice’s natural mechanisms for detoxifying cadmium were discussed. This study also intended to identify the absorption and transfer pathways of silicon and selenium in rice, their roles in improving rice structures, and their antagonistic effects on reducing cadmium stress (absorption, transport, and toxicity of cadmium).Keywords
Rice is the most consumed grain on the earth, which is the primary food source for more than 50% of the world’s population [1,2], and approximately 55%–80% of an individual’s calories come from rice, according to research [3]. The majority of people in many countries, such as China, eat rice as a staple food [4], and in Pakistan, rice is the second most consumed crop after wheat [5]. The population of the world will reach 9 billion by 2050, so rice production must also increase to ensure food security around the world, therefore scientists are always looking for ways to improve rice yields [6,7]. Recent changes in attitudes about food quality have led researchers to shift from quantitative to qualitative views of rice production, which in addition to producing high-yielding cultivars, maintains high standards for rice production, and directly improves human health [8–10].
The contamination of soils with heavy metals (such as lead, cadmium, nickel, and chromium) has become a major issue worldwide [1,11], which, in addition to reducing crop production, also increases crop contamination risks [1,12]. Cadmium is one of the most toxic heavy metals that is widely distributed throughout the environment today and has become a global concern [13,14]; Moreover, this element is extremely toxic to all organisms (plants, animals, and humans) in its ionic form [1,15]. Cd is also one of the most potential dangers to humans today due to its high stability, strong activity, and lack of decomposition by microbes [16,17]. Human activities such as mining, metal smelting, coal burning, and rock destruction contribute substantially to the pollution of Cd [18–20]; Additionally, Cd accumulation in the soil is caused by irrigation with sewage, sludge, long-term use of phosphate fertilizers, pesticides, and atmospheric deposition [20–22].
Studies have found that after lead (Pb) and mercury (Hg), Cd is the third most toxic metal in soil [7], and the US Environmental Protection Agency (EPA) and the Agency for Toxic Substances and Disease Registry (ATSDR) rank Cd seventh among the top 20 priority hazardous substances [23]. Additionally, studies in China have shown that 16.1% of soil samples collected throughout the country and 19.4% of arable land are contaminated with Cd [24]. The high bioavailability and extractability of Cd allow it to be easily absorbed by plant roots and other plant organs [20,25]; Therefore, Cd can enter the human food chain through plants, particularly grains, accumulate in the body, and endanger human health [16,26]. According to research, almost all food sources contain cadmium, meaning that most human populations are at risk [27], and because of Cd’s long half-life (30 years), it can accumulate in the body over time and cause irreversible damage [7,28]. The accumulation of Cd in the human body has been linked to diseases such as prostate cancer, lung cancer, osteoporosis, cardiovascular disease, kidney tubular necrosis, and neurotoxicity [29–32].
The national food safety standards of China (GB 2762-2020) made it illegal to sell products containing Cd at levels higher than 0.2 mg/kg [33]. However, it has been observed that plants contain Cd at levels ranging from 0.1 to 2.4 mg/kg dry weight (Table 1) [34]. Due to rice’s ability to absorb Cd from soil and transfer it to the seeds more efficiently than other grains [35], it is known as a Cd accumulator [14], which is the main source of Cd in dietary throughout the world [2]. Since rice is a staple food in most Asian countries and is an important tradable commodity worldwide [36,37], thus finding suitable solutions to reduce the effects of phytotoxic and Cd accumulation in rice grains can contribute significantly to human health [38,39].
Studies showed that Si is an effective technology for controlling Cd pollution in paddy soils, but its effectiveness depends on the dose, application method, and soil Si content [40]. Si also by improving soil ventilation, increasing soil pH, increasing the bioavailability of nutrients, and forming silicate complexes stabilizes Cd and reduce the extractable Cd for plants [13,16,41,42]. As shown by Ma et al., Si improves soil health without adversely affecting the soil bacterial community; the bacterial diversity is maintained, but the bacterial community’s structure is selectively changed, which reduces the risk of Cd contamination [16].
In several studies, it has been shown that low doses of Se can help plants grow and develop, as well as reduce biotic stress (such as plant diseases and pests) and abiotic stress (such as drought, heat, cold, salt, Ultraviolet radiation, heavy metals) for a wide range of plants [7,40,43,44]. Researchers have shown that Se can reduce rice’s absorption of heavy metals such as arsenic (As), Cd, mercury (Hg), lead (Pb), and antimony (Sb) [24,43]. In addition, the application of Se in different forms, such as soil application and foliar spraying, reduces the absorption and accumulation of heavy metals in rice [45,46]. The present study examines the molecular and morphophysiological mechanisms by which silicon and selenium reduce cadmium toxicity and accumulation in rice.
2 The Absorption, Transfer, and Effect of Cadmium (Cd) on Rice
Cd-contaminated soils cause physiological, morphological, biochemical, and molecular changes in rice [5,47], some of which will be discussed below. In addition to preventing seed germination and seedling growth, Cd causes a decrease in chlorophyll content and consequently a decrease in photosynthesis, damage to DNA, and dysfunction of some proteins, inhibiting the activity of enzymes involved in CO2 stabilization, leaf chlorosis, disruption of water balance, changes in carbohydrates, and ultimately inhibited rice growth and yield [15,35,38,48–50]. Additionally, Cd can inhibit the absorption of nutrients such as calcium (Ca), phosphorus (P), potassium (K), iron (Fe), zinc (Zn), and magnesium (Mg), resulting in nutrient imbalances in plants. [36,50,51]. Also, Cd creates reactive oxygen species (ROS) such as superoxide anion (O2−), hydrogen peroxide (H2O2), and hydroxyl free radical (OH−), raising malondialdehyde (MDA) levels, and affecting antioxidant enzymes [35,38,52].
Several studies have been conducted on the molecular mechanisms of Cd absorption and accumulation in rice, but in general, Cd transfer from soil to rice grain can be divided into five stages: 1) absorption from soil to symplast in root tissue, 2) sequestration in the root cells or flow to the xylem, 3) transport through the xylem, 4) transfer from xylem to phloem in nodes, 5) transfer through the phloem to the seeds [44,53,54]. Since Cd is absorbed by the roots, and the cell wall of the roots is the outermost protective layer of the cell against cadmium toxicity, identifying the pathways of Cd absorption and transfer in rice can greatly help to reduce the plant’s exposure to this heavy metal [12,55].
Researchers have found that OsNramp5, a member of the macrophage protein family associated with rice’s natural resistance, plays an important role in transferring cadmium from soil solution to root cells [15,56]. In addition, studies have found that OsHMA3 (heavy metal ATPase 3), a positional tonoplast transporter, transports Cd into root cell vacuoles [15,57]. In their studies, Nocito et al. observed that OSPC expression increased significantly in rice roots treated with Cd, suggesting this protein is involved in Cd transport to roots [58]. On the other hand, studies showed that OsHMA2, a homolog of OsHMA3, is located in the plasma membrane of onion epidermal root cells and is involved in the transfer of cadmium from roots to rice stems [15,45].
Cd must be transported from the roots to the rice grains through several nodes in the stem, where vascular bundles connect to the roots, leaves, and spikes [59]. During the transition of cadmium from the xylem to the phloem, the highest node in the stem plays a crucial role in accumulating this heavy metal in rice grains [59], As a result, increasing the inhibitory capacity of this node through agricultural methods or gene manipulation is crucial to reducing the accumulation of Cd in rice grains grown in soil contaminated with Cd [60]. There is evidence that the OsLCT1 and OsHMA2 genes are involved in the transfer of Cd from the phloem to the rice grains [15,61]; Moreover, Chen et al. showed that grains stored less Cd when OsLCT1 was knocked down in the uppermost nodes and leaf blades [60] (Fig. 1).
Figure 1: Genes involved in Cd uptake and translocation in rice. Nomenclature is as proposed by Ai et al. [76]
The largest amount of Cd stored in rice grains is transferred through the phloem and xylem during the grain-filling period [60,62]. In addition, during grain filling, the Cd deposited in the leaf blades is also transferred through the phloem to the grains after being re-directed in the nodes [46]. According to Rizwan et al. rice may accumulate excessive amounts of Cd as a result of increased tolerance due to chelation with organic acids and proteins, removal of Cd to tissues with less sensitivity, ionic homeostasis, the expression of different genes and increase plant growth regulators (PGR) [63].
In order to maintain natural rice growth and reduce human exposure to cadmium it is particularly important to reduce toxicity, transportation, and accumulation of cadmium in rice [5,45,64]. Cd is naturally reduced by several mechanisms in plants, including the following: 1) Chelating secretions such as organic acids with Cd and reducing the absorption of this heavy metal by roots [65], 2) reducing the apoplastic transfer of Cd in the xylem by creating exoderm and endoderm [66], 3) preventing symplastic transfer between cells by stabilizing Cd in cell walls and isolating it in vacuoles [35]. Although Cd increases ROS in tissues, however, rice naturally regulates it through low molecular weight substances like peroxidase (POD), superoxide dismutase (SOD), ascorbate peroxidase (APX), catalase (CAT), glutathione reductase (GR), glutathione peroxidase (GSH-Px), and ascorbic acid (AsA) [35,36,67].
Rice also detoxifies Cd through phytochelatin (PC) synthesis [68,69]; PC is a thiol-rich lipid that is synthesized from GSH by PC synthase (PCS) and has the general structure (γGlu-Cys)n-Gly (n = 2–12) [69]. PC can complex with Cd and reduce the amount of free Cd2+ within a cell, moreover, the PC-Cd complex is transported into vacuoles for storage [14,69]. In contrast to other cereal crops, rice has special aerenchyma that transports oxygen from the shoot to the roots, forming a micro-oxidation zone in the paddy soil [64,70]. In this case, Fe2+ ions in waterlogged soils can be oxidized to Fe3+ ions in the rhizosphere of rice roots, forming a red deposit known as iron plaques (IP) on the roots [70]. Research has shown that IP can adsorb cadmium on root surfaces and create a barrier to heavy metal uptake due to its large surface area for metal binding [71]. According to Gill and Tuteja, oxygen, which is one of the main products of ROS degradation, has a direct effect on radial oxygen loss (ROL) and IP formation [72]. Researchers have also focused on organic, inorganic, and farm management remediation methods to reduce cadmium accumulation in rice in recent years [59,73]. Among the proposed solutions are soil amendment, water management, and screening of rice varieties with low cadmium accumulation [41,74,75].
3 Silicon (Si) and Cadmium Antagonism in Rice
Silicon, the second most abundant element on earth, is found in all plants [77]; though it is not an essential element for plants, its absence causes irreparable damage to them and eventually to the agricultural economy [13,41]. Si accounts for approximately 10% of rice dry weight, making it a Si accumulator [36,40], and since Asian countries play an important role in rice production worldwide, due to the large cultivation of rice and the increase in temperatures have faced a lack of silica in the soil [36,78]. Furthermore, since most silicon in the soil is not bioavailable, it is necessary to use accessible forms of this element to improve rice production economically [79,80]. Besides adding Si fertilizers to paddy soil, solution spray is also effective for fertilizing with Si since the cuticles and stomas of the leaves absorb and internalize it [41,81]. The growth stage of rice also affects Si absorption efficiency, and according to research, the best time to apply Si fertilizer is during tillering and booting [41,82].
Si is absorbed and transferred through Lsi1, Lsi2, and Lsi6 genes in rice [47]; and high silicon content in rice tissues increases resistance against biotic and abiotic stress through physical and biochemical mechanisms [60,83]. Among various stresses, heavy metals are a major risk for rice cultivation, and several studies have shown that silicon reduces the risk of heavy metals like lead (Pb), arsenic (As), Cd, and chromium (Cr) in rice [1,13,45]. As a result, the Si application is one of the effective methods to reduce the absorption, transfer, and distribution of Cd in rice, which is an accessible and affordable solution [44,83,84]. Silicon reduces cadmium stress in rice in two general ways: internal (in planta) and external (ex planta) [83] and the effect of Si on reducing the risk of Cd will be discussed in more detail below.
Researchers on rice have found that Si can enhance the cell wall’s stability, which improves Cd’s absorption capacity into the endoderm wall, thus preventing apoplastic movement of Cd [16,50,60,85], and Si also reduces the free Cd content in cells by increasing chelation [16]. In addition, Chen et al. found that Si increases Cd segregation in vacuoles and prevents its mobility in plant tissues [60]. According to studies the addition of silicon in various forms reduces the transfer of Cd from the roots to the shoots [1,31,86], such that Shi et al. reported a 24% reduction in Cd content in rice shoots [87]. According to studies, Si foliar spraying significantly reduces Cd transfer from node I to internode I, which decreases Cd accumulation in the rice grain along with the root and node [59,60]. The researchers found that silicon is most effective in reducing cadmium levels during transplanting, tillering, and clustering [36]. As a result of studies conducted on the apoplasts and symplasts of rice roots, leaves, and stems, silicon application during transplanting has a greater impact on the cadmium content in the roots, whereas silicon application at the beginning of the cluster has a more effect on the cadmium accumulation in grains [41,50].
By adding Si to rice under Cd stress, cell division, cell length, carboxylation efficiency, chlorophyll content, transpiration efficiency, and gas exchange characteristics are improved [46,49,88,89], which ultimately increases plant photosynthesis, biomass, yields, and seed quality [41,90,91]. The application of Si in different forms, in addition to preventing the transfer of Cd from the roots to the aerial parts of rice, helps to improve the content of P, N, Ca, K, Mn, Zn, Fe, and Mg in rice organs, resulting in an improved plant nutrition [60,92]. Adding Si also enhances the ability to inhibit ROS generated by Cd stress by improving enzymatic and non-enzymatic activity mobility [47]. Si reduces oxidative stress in rice by improving the activities of POD, GSH, PC, SOD, CAT, APX, GPx, sucrose, and urease, as well as reducing MDA, OH−, O2−, H2O2, EL, NADPH oxidase, and lipid peroxidation in Cd-contaminated environments [16,31,45–47,93].
According to studies, Si increases the expression of approximately 50 proteins in rice seedlings grown in a Cd-contaminated medium [47]. A study by Lin et al. showed that Si stimulated the expression of the Lsi1 (OsNIP2;1) gene in roots of Cd-stressed rice, while OsHMA2, OsMHA3, and OsNramp5 were inhibited [47]. In their studies on rice, Huang et al. also demonstrated that silicon decreased the expression of the OsNramp1 gene, which is a cadmium transporter [45]. Under Cd stress, silicon alters the transcription of OsBTF3, Lhcb1, and RbcL genes and also improves the synthesis of psbA, Lhcb1, and RbcL proteins [44]. Additionally, Li et al. showed in their study that silicon reduced the toxicity of cadmium in rice by inhibiting YOR1 and YCF1 (transport-related genes) when the pH of contaminated soils was increased [94]. According to Wang et al., silicon reduces some genes expression in leaf (OsPCR1, OsHMA2, OsCCX2, and OsLCT1) and pedicle (OSPCR1, TaCNR2, OsCCX2, and OsLCT1) in Cd-stressed rice, which plays a role in the re-transfer of Cd from leaves or pedicels to seeds, thereby reducing Cd accumulation in rice grains [44].
4 Selenium (Se) and Cd Antagonism in Rice
Selenium is a vital micronutrient for humans and animals that plays an important role in a range of biological functions [38,95]. As Se reduces oxidative stress, prevents fertility decline, improves the immune system, improves cardiovascular health, and reduces cancer risk [43,96], and the recommended daily consumption of Se for adults is between 40 and 300 grams [7]. Since excessive levels or lack of Se can adversely affect human health, proper nutrition can reduce the risk of diseases in addition to absorbing the right amount of Se [97]. The most abundant source of Se for humans is cereals, and since rice is among the most popular foods, especially in Asia, research suggests it can meet almost the daily need for Se [43,98,99]. The element Se exists in nature in various forms, in addition to organic forms that can be easily absorbed by plant roots. It can be as Se (IV) which is the mold form of selenium in a well-drained mineral environment, as well as Se (VI) which is the main species in Alkaline and oxidized conditions [100]. Despite this, Se levels in the crust of the earth vary widely, such that 72% of Chinese land lacks Se, and 0.7 billion people worldwide suffer from Se deficiency, according to statistics [24,101].
Although Se is not an essential element for plants, however, it plays an important role in improving their physiological, morphological, and molecular structure if used in appropriate doses [38,102]. Plants absorb Se primarily from the soil, and several factors, including soil Se content, soil acidity, the form of Se in the soil, and the physical structure of the soil, influence this absorption [7]. The best plants for accumulating Se are generally cruciferous plants, legumes, and cereals [103]. Although 75% of rice samples examined in the world do not contain enough Se to satisfy human needs, the supply of Se required by these plants could contribute to improving human nutrition [98,103]. The use of Se nanoparticles (SeNPs) in rice nutrition has been investigated more in recent decades [104]; and since SeNPs have excellent bioavailability and high biological activity, they are less toxic than Se (IV) and Se (VI), and after absorption, rice converts them into Se (IV) and Se (VI) [105,106].
By a variety of mechanisms, Se reduces Cd absorption and accumulation in rice: 1) Reducing Cd’s bioavailability, 2) The regulation of antioxidant systems and metabolites, 3) Repair damaged cell membranes and regeneration of chloroplasts, 4) Increasing absorption of essential nutrients, and 5) Reducing expression of cadmium transporter genes [35,44,55]. In their studies, Huang et al. found that adding Se to Cd-contaminated soils, in addition to increasing soil pH, decreased the concentration of Cd in both the soil mass and the rhizosphere solution, which, in turn, reduced Cd’s bioavailability [43]. Furthermore, they found that Se increases Cd levels bound to carbonates and oxides of Fe and Mn, and therefore reduces exchangeable Cd in soil [43]; However, studies have shown that Se might reduce soil Cd availability, but may not reduce rice Cd absorption, and it depends on the characteristics of rice and the application time of Se [24,98,107].
Se increases rice root cell wall thickness, which increases mechanical strength, and a large amount of Cd accumulates there as a result [55]. In addition, Se increases the resistance of rice by partitioning Cd in vacuoles and creating the Se-Cd insoluble complex in the roots, preventing cadmium transfer to aerial organs [11,38,59,108]. According to Wan et al., both Se (IV) and Se (VI) reduced Cd translocation from the roots to shoots of rice seedlings [86], likely because Se reduces Cd loading in xylem sap [35]. In addition to reducing Cd absorption and transformation from root to shoot, Se also reduces Cd accumulation at nodes [38,59]; and due to the importance of the cluster node for allocating Cd to rice grains, Se cannot only increase the absorption of mineral nutrients in node I but also prevent the transfer of Cd from node I to the first internodes, and grain [59]. Besides the concentration, chemical form, and method of application of Se, the effectiveness of Se in reducing Cd risks is also dependent on the type of rice variety and stage of growth [24,29,107].
In environments contaminated with Cd, the addition of Se improves the recovery and reconstruction of cell structure (cell membranes and chloroplasts) [102] and enhances the efficiency of photosynthesis and respiration in rice seedlings [40,45,107], thus growth, development, and biomass production improves [38,107]. Additionally, selenium reduces the absorption and total level of cadmium in rice by increasing IP formation on root surfaces and reducing radial oxygen losses (ROL) [71]. According to previous studies, Se reduces Cd-induced oxidative stress by eliminating oxygen free radicals, reducing lipid peroxidation, and increasing beneficial antioxidant activity [43,109]. Studies have demonstrated that selenium increases the activities of POD, CAT, APX, SOD, and GPx, as well as the content of phytochelatin and GSH in rice cultivated in Cd-polluted environments [35,44,45,55] while decreasing the activity of NADPH oxidases and the content of MDA and H2O2 [38,44] (Fig. 2).
Figure 2: Protective roles of selenium on rice under cadmium stress. Se: Selenium. Cd: Cadmium. ROS: Reactive oxygen species. According to the graph, green or red represents factors that increased or decreased, respectively, with the addition of selenium in rice under cadmium stressed
Recent studies have shown that adding Se to Cd-contaminated cultures regulates the expression of genes involved in Cd absorption and transport to vacuoles in rice roots, including OsNramp1, OsNramp5, OsHMA2, and OsHMA3 [45,55]. Cui et al. found that the addition of Se increases the activity of genes involved in lignin synthesis (Os4CL3, OsCoMT, and OsPAL), leading to the increased thickness and mechanical strength of cell wall, thus increasing Cd fixation on the cell walls [55]. Researchers found that adding selenium reduces the expression of genes involved in Cd transfer in stressed rice leaves (OsPCR1, OsHMA2, OsCCX2, and OsLCT1) and stalks (OSPCR1, TaCNR2, OsCCX2, and OsLCT1) [44,55]. Further, Wang et al. found that selenium increases not only the transcription of the OsBTF3, RbcL, and Lhcb1 genes but also the production of 23 different proteins, including RbcL, Lhcb1, and psbA, which enhance chlorophyll content and RuBP carboxylase activity [44].
Based on the results of the study, silicon and selenium contribute to improving rice’s morphological, physiological, and biochemical characteristics, as well as its ability to resist cadmium stress. Additionally, the results indicated that the effectiveness of these elements in increasing resistance to cadmium stress depends on the growth stage and variety of rice as well as the doses and application methods. Several laboratory studies have investigated how silicon and selenium reduce cadmium stress in rice during recent decades. However, to identify their unknown effects on rice under cadmium stress, further research is needed, especially in field experiments and using different derivatives of these elements such as nanoparticles. Also, to improve the quantity and quality of rice produced and thereby enhance human health standards, more research is needed on the simultaneous application of silicon and selenium as well as the molecular effects of these elements on rice under cadmium stress in the coming years.
Funding Statement: This work was supported by the Anhui Province Large-Scale Online Open Course (MOOC) Demonstration Project (2018mooc428); the Quality Engineering Project of Suzhou University: College of Geographic Information and Energy Agriculture Modern Industry (szxy2021cyxy06); the Key Project of Quality Engineering in Anhui Province (2021jyxm1499); the Scientific Research Platform Project of Suzhou University: Research Center of Non-Point Source Pollution Control and Ecological Remediation (2021XJPT11).
Author Contributions: MRB wrote the manuscript. MRB and HYZ, HQ and JL edited the manuscript. All authors read and approved the final version of the manuscript.
Conflicts of Interest: The authors declare that they have no conflicts of interest to report regarding the present study.
References
1. Zaman, Q. U., Rashid, M., Nawaz, R., Hussain, A., Ashraf, K. et al. (2021). Silicon fertilization: A step towards cadmium-free fragrant rice. Plants, 10(11), 2440. https://doi.org/10.3390/plants10112440 [Google Scholar] [PubMed] [CrossRef]
2. Meharg, A. A., Norton, G., Deacon, C., Williams, P., Adomako, E. E. et al. (2013). Variation in rice cadmium related to human exposure. Environmental Science and Technology, 47(11), 5613–5618. https://doi.org/10.1021/es400521h [Google Scholar] [PubMed] [CrossRef]
3. Farooq, M., Basra, S. M. A., Wahid, A., Khaliq, A., Kobayashi, N. (2010). Rice seed invigoration: A review. Organic Farming, Pest Control and Remediation of Soil Pollutants, 1, 137–175. [Google Scholar]
4. Zhang, Y., Chen, T., Liao, Y., Reid, B. J., Chi, H. et al. (2016). Modest amendment of sewage sludge biochar to reduce the accumulation of cadmium into rice (Oryza sativa L.A field study. Environmental Pollution, 216(1), 819–825. https://doi.org/10.1016/j.envpol.2016.06.053 [Google Scholar] [PubMed] [CrossRef]
5. Ur Rehman, M. Z., Batool, Z., Ayub, M. A., Hussaini, K. M., Murtaza, G. et al. (2020). Effect of acidified biochar on bioaccumulation of cadmium (Cd) and rice growth in contaminated soil. Environmental Technology and Innovation, 19(1775), 101015. https://doi.org/10.1016/j.eti.2020.101015 [Google Scholar] [CrossRef]
6. Division, S. A. P. (2010). World population prospects, the 2008 revision. New York: United Nations Publications. [Google Scholar]
7. Farooq, M. U., Tang, Z., Zheng, T., Asghar, M. A., Zeng, R. et al. (2019). Cross-talk between cadmium and selenium at elevated cadmium stress determines the fate of selenium uptake in rice. Biomolecules, 9(6), 247. https://doi.org/10.3390/biom9060247 [Google Scholar] [PubMed] [CrossRef]
8. Ellis, D. R., Salt, D. E. (2003). Plants, selenium and human health. Current Opinion in Plant Biology, 6(3), 273–279. https://doi.org/10.1016/S1369-5266(03)00030-X [Google Scholar] [PubMed] [CrossRef]
9. Liu, W., Oosterveer, P., Spaargaren, G. (2016). Promoting sustainable consumption in China: A conceptual framework and research review. Journal of Cleaner Production, 134(6), 13–21. https://doi.org/10.1016/j.jclepro.2015.10.124 [Google Scholar] [CrossRef]
10. Yan, F., Sun, Y., Xu, H., Yin, Y., Wang, H. et al. (2018). Effects of wheat straw mulch application and nitrogen management on rice root growth, dry matter accumulation and rice quality in soils of different fertility. Paddy and Water Environment, 16(3), 507–518. https://doi.org/10.1007/s10333-018-0643-1 [Google Scholar] [CrossRef]
11. Zheng, H., Wang, M., Chen, S., Li, S., Lei, X. (2019). Sulfur application modifies cadmium availability and transfer in the soil-rice system under unstable pe+pH conditions. Ecotoxicology and Environmental Safety, 184(24), 109641. https://doi.org/10.1016/j.ecoenv.2019.109641 [Google Scholar] [PubMed] [CrossRef]
12. Hafeez, F., Rizwan, M., Saqib, M., Yasmeen, T., Ali, S. et al. (2019). Residual effect of biochar on growth, antioxidant defence and cadmium (Cd) accumulation in rice in a Cd contaminated saline soil. Pakistan Journal of Agricultural Sciences, 56, 197–204. [Google Scholar]
13. Zhao, F. J., Wang, P. (2020). Arsenic and cadmium accumulation in rice and mitigation strategies. Plant and Soil, 446(1–2), 1–21. https://doi.org/10.1007/s11104-019-04374-6 [Google Scholar] [CrossRef]
14. Chen, J., Huang, X. Y., Salt, D. E., Zhao, F. J. (2020). Mutation in OsCADT1 enhances cadmium tolerance and enriches selenium in rice grain. New Phytologist, 226(3), 838–850. https://doi.org/10.1111/nph.16404 [Google Scholar] [PubMed] [CrossRef]
15. Feng, S. J., Che, J., Yamaji, N., Fang, S. R., Feng, M. J. (2017). Silicon reduces cadmium accumulation by suppressing expression of transporter genes involved in cadmium uptake and translocation in rice. Journal of Experimental Botany, 68(20), 5641–5651. https://doi.org/10.1093/jxb/erx364 [Google Scholar] [PubMed] [CrossRef]
16. Ma, C., Ci, K., Zhu, J., Sun, Z., Liu, Z. et al. (2021). Impacts of exogenous mineral silicon on cadmium migration and transformation in the soil-rice system and on soil health. Science of the Total Environment, 759(1–8), 143501. https://doi.org/10.1016/j.scitotenv.2020.143501 [Google Scholar] [PubMed] [CrossRef]
17. Yao, A., Liu, Y., Luo, X., Liu, C., Tang, Y. et al. (2021). Mediation effects of different sulfur forms on solubility, uptake and accumulation of Cd in soil-paddy rice system induced by organic carbon and liming. Environmental Pollution, 279(8), 116862. https://doi.org/10.1016/j.envpol.2021.116862 [Google Scholar] [PubMed] [CrossRef]
18. Rizwan, M., Ali, S., Abbas, T., Adrees, M., Zia-ur-Rehman, M. et al. (2018). Residual effects of biochar on growth, photosynthesis and cadmium uptake in rice (Oryza sativa L.) under Cd stress with different water conditions. Journal of Environmental Management, 206, 676–683. https://doi.org/10.1016/j.jenvman.2017.10.035 [Google Scholar] [PubMed] [CrossRef]
19. Zhao, F. J., Ma, Y., Zhu, Y. G., Tang, Z., McGrath, S. P. (2015). Soil contamination in China: Current status and mitigation strategies. Environmental Science and Technology, 49(2), 750–759. https://doi.org/10.1021/es5047099 [Google Scholar] [PubMed] [CrossRef]
20. Li, H., Luo, N., Li, Y. W., Cai, Q. Y., Li, H. Y. et al. (2017). Cadmium in rice: Transport mechanisms, influencing factors, and minimizing measures. Environmental Pollution, 224(6), 622–630. https://doi.org/10.1016/j.envpol.2017.01.087 [Google Scholar] [PubMed] [CrossRef]
21. Yi, K., Fan, W., Chen, J., Jiang, S., Huang, S. et al. (2018). Annual input and output fluxes of heavy metals to paddy fields in four types of contaminated areas in Hunan Province, China. Science of the Total Environment, 634, 67–76. https://doi.org/10.1016/j.scitotenv.2018.03.294 [Google Scholar] [PubMed] [CrossRef]
22. Herawati, N., Suzuki, S., Hayashi, K., Rivai, I., Koyama, H. (2000). Cadmium, copper, and zinc levels in rice and soil of Japan, Indonesia, and China by soil type. Bulletin of Environmental Contamination and Toxicology, 64(1), 33–39. https://doi.org/10.1007/s001289910006 [Google Scholar] [PubMed] [CrossRef]
23. Yang, X., Long, X., Ye, H., He, Z., Calvert, D. et al. (2004). Cadmium tolerance and hyperaccumulation in a new Zn-hyperaccumulating plant species (Sedum alfredii Hance). Plant and Soil, 259(1/2), 181–189. https://doi.org/10.1023/B:PLSO.0000020956.24027.f2 [Google Scholar] [CrossRef]
24. Yang, B. B., Yang, C., Shao, Z. Y., Wang, H., Zan, S. T. et al. (2019). Selenium (Se) does not reduce cadmium (Cd) uptake and translocation in rice (Oryza sativa L.) in naturally occurred Se-rich paddy fields with a high geological background of Cd. Bulletin of Environmental Contamination and Toxicology, 103(1), 127–132. https://doi.org/10.1007/s00128-019-02551-y [Google Scholar] [PubMed] [CrossRef]
25. Liu, X., Zhong, L., Meng, J., Wang, F., Zhang, J. et al. (2018). A multi-medium chain modeling approach to estimate the cumulative effects of cadmium pollution on human health. Environmental Pollution, 239(1–3), 308–317. https://doi.org/10.1016/j.envpol.2018.04.033 [Google Scholar] [PubMed] [CrossRef]
26. Clemens, S., Aarts, M. G., Thomine, S., Verbruggen, N. (2013). Plant science: The key to preventing slow cadmium poisoning. Trends in Plant Science, 18(2), 92–99. https://doi.org/10.1016/j.tplants.2012.08.003 [Google Scholar] [PubMed] [CrossRef]
27. Nawrot, T., Plusquin, M., Hogervorst, J., Roels, H. A., Celis, H. et al. (2006). Environmental exposure to cadmium and risk of cancer: A prospective population-based study. The Lancet Oncology, 7(2), 119–126. https://doi.org/10.1016/S1470-2045(06)70545-9 [Google Scholar] [PubMed] [CrossRef]
28. Ismael, M. A., Elyamine, A. M., Moussa, M. G., Cai, M., Zhao, X. et al. (2019). Cadmium in plants: Uptake, toxicity, and its interactions with selenium fertilizers. Metallomics, 11(2), 255–277. https://doi.org/10.1039/C8MT00247A [Google Scholar] [PubMed] [CrossRef]
29. Yang, R., He, Y., Luo, L., Zhu, M., Zan, S. et al. (2021). The interaction between selenium and cadmium in the soil-rice-human continuum in an area with high geological background of selenium and cadmium. Ecotoxicology and Environmental Safety, 222(688), 112516. https://doi.org/10.1016/j.ecoenv.2021.112516 [Google Scholar] [PubMed] [CrossRef]
30. Sun, L., Song, K., Shi, L., Duan, D., Zhang, H. et al. (2021). Influence of elemental sulfur on cadmium bioavailability, microbial community in paddy soil and Cd accumulation in rice plants. Scientific Reports, 11(1), 1–9. https://doi.org/10.1038/s41598-021-91003-x [Google Scholar] [PubMed] [CrossRef]
31. Chen, D., Chen, D., Xue, R., Long, J., Lin, X. et al. (2019). Effects of boron, silicon and their interactions on cadmium accumulation and toxicity in rice plants. Journal of Hazardous Materials, 367, 447–455. https://doi.org/10.1016/j.jhazmat.2018.12.111 [Google Scholar] [PubMed] [CrossRef]
32. Zhang, W. L., Du, Y., Zhai, M. M., Shang, Q. (2014). Cadmium exposure and its health effects: A 19-year follow-up study of a polluted area in China. Science of the Total Environment, 470(5), 224–228. https://doi.org/10.1016/j.scitotenv.2013.09.070 [Google Scholar] [PubMed] [CrossRef]
33. Hussain, S., Yang, J. J., Hussain, J., Zandi, P., Xing, X. et al. (2022). The rhizospheric transformation and bioavailability of mercury in pepper plants are influenced by selected Chinese soil types. Environmental Geochemistry and Health, 45(7), 1–12. [Google Scholar]
34. Boorboori, M. R., Zhang, H. Y. (2022). Arbuscular mycorrhizal fungi are an influential factor in improving the phytoremediation of arsenic, cadmium, lead, and chromium. Journal of Fungi, 8, 176. [Google Scholar] [PubMed]
35. Wan, Y., Wang, K., Liu, Z., Yu, Y., Wang, Q. et al. (2019). Effect of selenium on the subcellular distribution of cadmium and oxidative stress induced by cadmium in rice (Oryza sativa L.). Environmental Science and Pollution Research, 26(16), 16220–16228. https://doi.org/10.1007/s11356-019-04975-9 [Google Scholar] [PubMed] [CrossRef]
36. Ur Rehman, M. Z., Rizwan, M., Rauf, A., Ayub, M. A., Ali, S. et al. (2019). Split application of silicon in cadmium (Cd) spiked alkaline soil plays a vital role in decreasing Cd accumulation in rice (Oryza sativa L.) grains. Chemosphere, 226, 454–462. https://doi.org/10.1016/j.chemosphere.2019.03.182 [Google Scholar] [PubMed] [CrossRef]
37. Honma, T., Ohba, H., Kaneko-Kadokura, A., Makino, T., Nakamura, K. et al. (2016). Optimal soil Eh, pH, and water management for simultaneously minimizing arsenic and cadmium concentrations in rice grains. Environmental Science & Technology, 50(8), 4178–4185. https://doi.org/10.1021/acs.est.5b05424 [Google Scholar] [PubMed] [CrossRef]
38. Xu, H., Yan, J., Qin, Y., Xu, J., Shohag, M. et al. (2020). Effect of different forms of selenium on the physiological response and the cadmium uptake by rice under cadmium stress. International Journal of Environmental Research and Public Health, 17(19), 6991. https://doi.org/10.3390/ijerph17196991 [Google Scholar] [PubMed] [CrossRef]
39. Luo, J. S., Huang, J., Zeng, D. L., Peng, J. S., Zhang, G. B. et al. (2018). A defensin-like protein drives cadmium efflux and allocation in rice. Nature Communications, 9(1), 1–9. https://doi.org/10.1038/s41467-018-03088-0 [Google Scholar] [PubMed] [CrossRef]
40. Li, N., Feng, A., Liu, N., Jiang, Z., Wei, S. (2020). Silicon application improved the yield and nutritional quality while reduced cadmium concentration in rice. Environmental Science and Pollution Research, 27(16), 20370–20379. https://doi.org/10.1007/s11356-020-08357-4 [Google Scholar] [PubMed] [CrossRef]
41. Cai, Y., Zhang, S., Cai, K., Huang, F., Pan, B. et al. (2020). Cd accumulation, biomass and yield of rice are varied with silicon application at different growth phases under high concentration cadmium-contaminated soil. Chemosphere, 242, 125128. https://doi.org/10.1016/j.chemosphere.2019.125128 [Google Scholar] [PubMed] [CrossRef]
42. Liang, Y., Wong, J., Wei, L. (2005). Silicon-mediated enhancement of cadmium tolerance in maize (Zea mays L.) grown in cadmium contaminated soil. Chemosphere, 58(4), 475–483. https://doi.org/10.1016/j.chemosphere.2004.09.034 [Google Scholar] [PubMed] [CrossRef]
43. Huang, Q., Xu, Y., Liu, Y., Qin, X., Huang, R. et al. (2018). Selenium application alters soil cadmium bioavailability and reduces its accumulation in rice grown in Cd-contaminated soil. Environmental Science and Pollution Research, 25(31), 31175–31182. https://doi.org/10.1007/s11356-018-3068-x [Google Scholar] [PubMed] [CrossRef]
44. Wang, C., Rong, H., Zhang, X., Shi, W., Hong, X. et al. (2020). Effects and mechanisms of foliar application of silicon and selenium composite sols on diminishing cadmium and lead translocation and affiliated physiological and biochemical responses in hybrid rice (Oryza sativa L.) exposed to cadmium and lead. Chemosphere, 251(4), 126347. https://doi.org/10.1016/j.chemosphere.2020.126347 [Google Scholar] [PubMed] [CrossRef]
45. Huang, H., Li, M., Rizwan, M., Dai, Z., Yuan, Y. et al. (2021). Synergistic effect of silicon and selenium on the alleviation of cadmium toxicity in rice plants. Journal of Hazardous Materials, 401(6), 123393. https://doi.org/10.1016/j.jhazmat.2020.123393 [Google Scholar] [PubMed] [CrossRef]
46. Wang, Y., Luo, W., Zeng, G., Peng, H., Cheng, A. et al. (2020). Characteristics of carbon, water, and energy fluxes on abandoned farmland revealed by critical zone observation in the Karst region of Southwest China. Agriculture, Ecosystems and Environment, 292(1), 106821. https://doi.org/10.1016/j.agee.2020.106821 [Google Scholar] [CrossRef]
47. Lin, H., Fang, C., Li, Y., Lin, W., He, J. et al. (2017). Cadmium-stress mitigation through gene expression of rice and silicon addition. Plant Growth Regulation, 81(1), 91–101. https://doi.org/10.1007/s10725-016-0191-x [Google Scholar] [CrossRef]
48. Demim, S., Drouiche, N., Aouabed, A., Benayad, T., Dendene-Badache, O. et al. (2013). Assessment of the physiological effects and heavy metal removal using a response surface approach by L. gibba. Ecological Engineering, 61(8), 426–435. https://doi.org/10.1016/j.ecoleng.2013.10.016 [Google Scholar] [CrossRef]
49. Gao, M., Zhou, J., Liu, H., Zhang, W., Hu, Y. et al. (2018). Foliar spraying with silicon and selenium reduces cadmium uptake and mitigates cadmium toxicity in rice. Science of the Total Environment, 631(46), 1100–1108. https://doi.org/10.1016/j.scitotenv.2018.03.047 [Google Scholar] [PubMed] [CrossRef]
50. Ji, X., Liu, S., Juan, H., Bocharnikova, E. A., Matichenkov, V. V. (2017). Effect of silicon fertilizers on cadmium in rice (Oryza sativa) tissue at tillering stage. Environmental Science and Pollution Research, 24(11), 10740–10748. https://doi.org/10.1007/s11356-017-8730-1 [Google Scholar] [PubMed] [CrossRef]
51. DalCorso, G., Farinati, S., Maistri, S., Furini, A. (2008). How plants cope with cadmium: Staking all on metabolism and gene expression. Journal of Integrative Plant Biology, 50(10), 1268–1280. https://doi.org/10.1111/j.1744-7909.2008.00737.x [Google Scholar] [PubMed] [CrossRef]
52. Rizwan, M., Ali, S., Malik, S., Adrees, M., Qayyum, M. F. (2019). Effect of foliar applications of silicon and titanium dioxide nanoparticles on growth, oxidative stress, and cadmium accumulation by rice (Oryza sativa L.). Acta Physiologiae Plantarum, 41, 1–12. [Google Scholar]
53. Clemens, S., Ma, J. F. (2016). Toxic heavy metal and metalloid accumulation in crop plants and foods. Annual Review of Plant Biology, 67(1), 489–512. https://doi.org/10.1146/annurev-arplant-043015-112301 [Google Scholar] [PubMed] [CrossRef]
54. Fujimaki, S., Suzui, N., Ishioka, N. S., Kawachi, N., Ito, S. et al. (2010). Tracing cadmium from culture to spikelet: Noninvasive imaging and quantitative characterization of absorption, transport, and accumulation of cadmium in an intact rice plant. Plant Physiology, 152(4), 1796–1806. https://doi.org/10.1104/pp.109.151035 [Google Scholar] [PubMed] [CrossRef]
55. Cui, J., Liu, T., Li, Y., Li, F. (2018). Selenium reduces cadmium uptake into rice suspension cells by regulating the expression of lignin synthesis and cadmium-related genes. Science of the Total Environment, 644, 602–610. https://doi.org/10.1016/j.scitotenv.2018.07.002 [Google Scholar] [PubMed] [CrossRef]
56. Uraguchi, S., Fujiwara, T. (2013). Rice breaks ground for cadmium-free cereals. Current Opinion in Plant Biology, 16(3), 328–334. https://doi.org/10.1016/j.pbi.2013.03.012 [Google Scholar] [PubMed] [CrossRef]
57. Sasaki, A., Yamaji, N., Ma, J. F. (2014). Overexpression of OsHMA3 enhances Cd tolerance and expression of Zn transporter genes in rice. Journal of Experimental Botany, 65(20), 6013–6021. https://doi.org/10.1093/jxb/eru340 [Google Scholar] [PubMed] [CrossRef]
58. Nocito, F. F., Lancilli, C., Dendena, B., Lucchini, G., Sacchi, G. A. (2011). Cadmium retention in rice roots is influenced by cadmium availability, chelation and translocation. Plant, Cell and Environment, 34(6), 994–1008. https://doi.org/10.1111/j.1365-3040.2011.02299.x [Google Scholar] [PubMed] [CrossRef]
59. Zhou, J., Gao, M., Cui, H., Li, D., Xia, R. et al. (2021). Influence of silicon and selenium and contribution of the node to cadmium allocation and toxicity in rice. ACS Agricultural Science and Technology, 1(5), 550–557. https://doi.org/10.1021/acsagscitech.1c00157 [Google Scholar] [CrossRef]
60. Chen, R., Zhang, C., Zhao, Y., Huang, Y., Liu, Z. (2018). Foliar application with nano-silicon reduced cadmium accumulation in grains by inhibiting cadmium translocation in rice plants. Environmental Science and Pollution Research, 25(3), 2361–2368. https://doi.org/10.1007/s11356-017-0681-z [Google Scholar] [PubMed] [CrossRef]
61. Uraguchi, S., Kamiya, T., Sakamoto, T., Kasai, K., Sato, Y. et al. (2011). Low-affinity cation transporter (OsLCT1) regulates cadmium transport into rice grains. Proceedings of The National Academy of Sciences of the United States of America, 108(52), 20959–20964. https://doi.org/10.1073/pnas.1116531109 [Google Scholar] [PubMed] [CrossRef]
62. Zhou, H., Zhu, W., Yang, W. T., Gu, J. F., Gao, Z. X. et al. (2018). Cadmium uptake, accumulation, and remobilization in iron plaque and rice tissues at different growth stages. Ecotoxicology and Environmental Safety, 152, 91–97. https://doi.org/10.1016/j.ecoenv.2018.01.031 [Google Scholar] [PubMed] [CrossRef]
63. Rizwan, M., Ali, S., Adrees, M., Rizvi, H., Zia-ur-Rehman, M. et al. (2016). Cadmium stress in rice: Toxic effects, tolerance mechanisms, and management: A critical review. Environmental Science and Pollution Research, 23(18), 17859–17879. https://doi.org/10.1007/s11356-016-6436-4 [Google Scholar] [PubMed] [CrossRef]
64. Bailey-Serres, J., Lee, S. C., Brinton, E. (2012). Waterproofing crops: Effective flooding survival strategies. Plant Physiology, 160(4), 1698–1709. https://doi.org/10.1104/pp.112.208173 [Google Scholar] [PubMed] [CrossRef]
65. Dong, J., Mao, W., Zhang, G., Wu, F., Cai, Y. (2007). Root excretion and plant tolerance to cadmium toxicity–A review. Plant Soil and Environment, 53(5), 193–200. https://doi.org/10.17221/2205-PSE [Google Scholar] [CrossRef]
66. Lux, A., Martinka, M., Vaculík, M., White, P. J. (2011). Root responses to cadmium in the rhizosphere: A review. Journal of Experimental Botany, 62(1), 21–37. https://doi.org/10.1093/jxb/erq281 [Google Scholar] [PubMed] [CrossRef]
67. Liu, Y., Zhang, C., Zhao, Y., Sun, S., Liu, Z. (2017). Effects of growing seasons and genotypes on the accumulation of cadmium and mineral nutrients in rice grown in cadmium contaminated soil. Science of the Total Environment, 579(3), 1282–1288. https://doi.org/10.1016/j.scitotenv.2016.11.115 [Google Scholar] [PubMed] [CrossRef]
68. Uraguchi, S., Tanaka, N., Hofmann, C., Abiko, K., Ohkama-Ohtsu, N. et al. (2017). Phytochelatin synthase has contrasting effects on cadmium and arsenic accumulation in rice grains. Plant and Cell Physiology, 58(10), 1730–1742. https://doi.org/10.1093/pcp/pcx114 [Google Scholar] [PubMed] [CrossRef]
69. Cobbett, C., Goldsbrough, P. (2002). Phytochelatins AND metallothioneins: Roles. Annual Review of Plant Biology, 53(1), 159–182. https://doi.org/10.1146/annurev.arplant.53.100301.135154 [Google Scholar] [PubMed] [CrossRef]
70. Liu, W., Zhu, Y., Hu, Y., Williams, P., Gault, A. et al. (2006). Arsenic sequestration in iron plaque, its accumulation and speciation in mature rice plants (Oryza sativa L.). Environmental Science and Technology, 40(18), 5730–5736. https://doi.org/10.1021/es060800v [Google Scholar] [PubMed] [CrossRef]
71. Huang, G., Ding, C., Li, Y., Zhang, T., Wang, X. (2020). Selenium enhances iron plaque formation by elevating the radial oxygen loss of roots to reduce cadmium accumulation in rice (Oryza sativa L.). Journal of Hazardous Materials, 398, 122860. https://doi.org/10.1016/j.jhazmat.2020.122860 [Google Scholar] [PubMed] [CrossRef]
72. Gill, S. S., Tuteja, N. (2010). Reactive oxygen species and antioxidant machinery in abiotic stress tolerance in crop plants. Plant Physiology and Biochemistry, 48(12), 909–930. https://doi.org/10.1016/j.plaphy.2010.08.016 [Google Scholar] [PubMed] [CrossRef]
73. Zhang, D., Du, G., Chen, D., Shi, G., Rao, W. et al. (2019). Effect of elemental sulfur and gypsum application on the bioavailability and redistribution of cadmium during rice growth. Science of the Total Environment, 657(10), 1460–1467. https://doi.org/10.1016/j.scitotenv.2018.12.057 [Google Scholar] [PubMed] [CrossRef]
74. Tang, X., Li, Q., Wu, M., Lin, L., Scholz, M. (2016). Review of remediation practices regarding cadmium-enriched farmland soil with particular reference to China. Journal of Environmental Management, 181, 646–662. https://doi.org/10.1016/j.jenvman.2016.08.043 [Google Scholar] [PubMed] [CrossRef]
75. Cai, Y., Wang, X., Beesley, L., Zhang, Z., Zhi, S. et al. (2021). Cadmium uptake reduction in paddy rice with a combination of water management, soil application of calcium magnesium phosphate and foliar spraying of Si/Se. Environmental Science and Pollution Research, 28(36), 50378–50387. https://doi.org/10.1007/s11356-021-13512-6 [Google Scholar] [PubMed] [CrossRef]
76. Ai, H., Wu, D., Li, C., Hou, M. (2022). Advances in molecular mechanisms underlying cadmium uptake and translocation in rice. Frontiers in Plant Science, 13, 431. https://doi.org/10.3389/fpls.2022.1003953 [Google Scholar] [PubMed] [CrossRef]
77. Ma, J. F., Tamai, K., Yamaji, N., Mitani, N., Konishi, S. et al. (2006). A silicon transporter in rice. Nature, 440(7084), 688–691. https://doi.org/10.1038/nature04590 [Google Scholar] [PubMed] [CrossRef]
78. Klotzbücher, T., Marxen, A., Vetterlein, D., Schneiker, J., Türke, M. et al. (2015). Plant-available silicon in paddy soils as a key factor for sustainable rice production in Southeast Asia. Basic and Applied Ecology, 16(8), 665–673. https://doi.org/10.1016/j.baae.2014.08.002 [Google Scholar] [CrossRef]
79. Pukto, P., Kwaśny, M. (2020). Bioavailable silicon forms in dietary supplements. Biuletyn Wojskowej Akademii Technicznej, 69, 35–41. [Google Scholar]
80. Ning, D., Song, A., Fan, F., Li, Z., Liang, Y. (2014). Effects of slag-based silicon fertilizer on rice growth and brown-spot resistance. PLoS One, 9(7), 102681. https://doi.org/10.1371/journal.pone.0102681 [Google Scholar] [PubMed] [CrossRef]
81. Shahid, M., Dumat, C., Khalid, S., Schreck, E., Xiong, T. et al. (2017). Foliar heavy metal uptake, toxicity and detoxification in plants: A comparison of foliar and root metal uptake. Journal of Hazardous Materials, 325, 36–58. https://doi.org/10.1016/j.jhazmat.2016.11.063 [Google Scholar] [PubMed] [CrossRef]
82. Gan, X. Q., Jiang, L. G., Xu, J. Y., Dong, D. F., Wei, S. Q. (2004). Characteristics and genotypic difference of silicon accumulation and distribution in rice. Plant Nutrition and Fertitizer Science, 10, 531–535. [Google Scholar]
83. Xiao, Z., Peng, M., Mei, Y., Tan, L., Liang, Y. et al. (2021). Effect of organosilicone and mineral silicon fertilizers on chemical forms of cadmium and lead in soil and their accumulation in rice. Environmental Pollution, 283, 117107. https://doi.org/10.1016/j.envpol.2021.117107 [Google Scholar] [PubMed] [CrossRef]
84. Yu, H. Y., Ding, X., Li, F., Wang, X., Zhang, S. (2016). The availabilities of arsenic and cadmium in rice paddy fields from a mining area: The role of soil extractable and plant silicon. Environmental Pollution, 215, 258–265. https://doi.org/10.1016/j.envpol.2016.04.008 [Google Scholar] [PubMed] [CrossRef]
85. Ma, J., Cai, H., He, C., Zhang, W., Wang, L. (2015). A hemicellulose-bound form of silicon inhibits cadmium ion uptake in rice (Oryza sativa) cells. New Phytologist, 206(3), 1063–1074. https://doi.org/10.1111/nph.13276 [Google Scholar] [PubMed] [CrossRef]
86. Wan, Y., Yu, Y., Wang, Q., Qiao, Y., Li, H. (2016). Cadmium uptake dynamics and translocation in rice seedling: Influence of different forms of selenium. Ecotoxicology and Environmental Safety, 133(6), 127–134. https://doi.org/10.1016/j.ecoenv.2016.07.001 [Google Scholar] [PubMed] [CrossRef]
87. Shi, X., Zhang, C., Wang, H., Zhang, F. (2005). Effect of Si on the distribution of Cd in rice seedlings. Plant and Soil, 272(1–2), 53–60. https://doi.org/10.1007/s11104-004-3920-2 [Google Scholar] [CrossRef]
88. Shireen, F., Nawaz, M. A., Chen, C., Zhang, Q., Zheng, Z. et al. (2018). Boron: Functions and approaches to enhance its availability in plants for sustainable agriculture. International Journal of Molecular Sciences, 19(7), 1856. https://doi.org/10.3390/ijms19071856 [Google Scholar] [PubMed] [CrossRef]
89. Adrees, M., Ali, S., Rizwan, M., Zia-ur-Rehman, M., Ibrahim, M. et al. (2015). Mechanisms of silicon-mediated alleviation of heavy metal toxicity in plants: A review. Ecotoxicology and Environmental Safety, 119(11), 186–197. https://doi.org/10.1016/j.ecoenv.2015.05.011 [Google Scholar] [PubMed] [CrossRef]
90. Lavinsky, A. O., Detmann, K. C., Reis, J. V., Ávila, R. T., Sanglard, M. L. et al. (2016). Silicon improves rice grain yield and photosynthesis specifically when supplied during the reproductive growth stage. Journal of Plant Physiology, 206, 125–132. https://doi.org/10.1016/j.jplph.2016.09.010 [Google Scholar] [PubMed] [CrossRef]
91. Meharg, C., Meharg, A. A. (2015). Silicon, the silver bullet for mitigating biotic and abiotic stress, and improving grain quality, in rice? Environmental and Experimental Botany, 120(1), 8–17. https://doi.org/10.1016/j.envexpbot.2015.07.001 [Google Scholar] [CrossRef]
92. Hernandez-Apaolaza, L. (2014). Can silicon partially alleviate micronutrient deficiency in plants? A review. Planta, 240(3), 447–458. https://doi.org/10.1007/s00425-014-2119-x [Google Scholar] [PubMed] [CrossRef]
93. Alscher, R. G., Erturk, N., Heath, L. S. (2002). Role of superoxide dismutases (SODs) in controlling oxidative stress in plants. Journal of Experimental Botany, 53(372), 1331–1341. https://doi.org/10.1093/jexbot/53.372.1331 [Google Scholar] [CrossRef]
94. Li, C., Yang, X., Xu, Y., Li, L., Wang, Y. (2018). Cadmium detoxification induced by salt stress improves cadmium tolerance of multi-stress-tolerant Pichia kudriavzevii. Environmental Pollution, 242, 845–854. https://doi.org/10.1016/j.envpol.2018.07.058 [Google Scholar] [PubMed] [CrossRef]
95. Brown, K. M., Arthur, J. (2001). Selenium, selenoproteins and human health: A review. Public Health Nutrition, 4(2b), 593–599. https://doi.org/10.1079/PHN2001143 [Google Scholar] [PubMed] [CrossRef]
96. Rayman, M. P. (2000). The importance of selenium to human health. The Lancet, 356(9225), 233–241. https://doi.org/10.1016/S0140-6736(00)02490-9 [Google Scholar] [PubMed] [CrossRef]
97. Bouayed, J., Bohn, T. (2010). Exogenous antioxidants—double-edged swords in cellular redox state: Health beneficial effects at physiologic doses versus deleterious effects at high doses. Oxidative Medicine and Cellular Longevity, 3(4), 228–237. https://doi.org/10.4161/oxim.3.4.12858 [Google Scholar] [PubMed] [CrossRef]
98. Guo, Y., Mao, K., Cao, H., Ali, W., Lei, D. et al. (2021). Exogenous selenium (cadmium) inhibits the absorption and transportation of cadmium (selenium) in rice. Environmental Pollution, 268, 115829. https://doi.org/10.1016/j.envpol.2020.115829 [Google Scholar] [PubMed] [CrossRef]
99. Zhang, H., Feng, X., Jiang, C., Li, Q., Liu, Y. et al. (2014). Understanding the paradox of selenium contamination in mercury mining areas: High soil content and low accumulation in rice. Environmental Pollution, 188, 27–36. https://doi.org/10.1016/j.envpol.2014.01.012 [Google Scholar] [PubMed] [CrossRef]
100. Kikkert, J., Berkelaar, E. (2013). Plant uptake and translocation of inorganic and organic forms of selenium. Archives of Environmental Contamination and Toxicology, 65(3), 458–465. https://doi.org/10.1007/s00244-013-9926-0 [Google Scholar] [PubMed] [CrossRef]
101. Gao, J., Liu, Y., Huang, Y., Lin, Z. Q., Bañuelos, G. S. et al. (2011). Daily selenium intake in a moderate selenium deficiency area of Suzhou. China Food Chemistry, 126(3), 1088–1093. https://doi.org/10.1016/j.foodchem.2010.11.137 [Google Scholar] [CrossRef]
102. Feng, R., Wei, C., Tu, S. (2013). The roles of selenium in protecting plants against abiotic stresses. Environmental and Experimental Botany, 87(2), 58–68. https://doi.org/10.1016/j.envexpbot.2012.09.002 [Google Scholar] [CrossRef]
103. Williams, P. N., Lombi, E., Sun, G. X., Scheckel, K., Zhu, Y. G. et al. (2009). Selenium characterization in the global rice supply chain. Environmental Science and Technology, 43(15), 6024–6030. https://doi.org/10.1021/es900671m [Google Scholar] [PubMed] [CrossRef]
104. Li, Y., Zhu, N., Liang, X., Zheng, L., Zhang, C. et al. (2020). A comparative study on the accumulation, translocation and transformation of selenite, selenate, and SeNPs in a hydroponic-plant system. Ecotoxicology and Environmental Safety, 189(1), 109955. https://doi.org/10.1016/j.ecoenv.2019.109955 [Google Scholar] [PubMed] [CrossRef]
105. El-Ramady, H., Abdalla, N., Taha, H. S., Alshaal, T., El-Henawy, A. et al. (2016). Selenium and nano-selenium in plant nutrition. Environmental Chemistry Letters, 14(1), 123–147. https://doi.org/10.1007/s10311-015-0535-1 [Google Scholar] [CrossRef]
106. Hu, T., Li, H., Li, J., Zhao, G., Wu, W. et al. (2018). Absorption and bio-transformation of selenium nanoparticles by wheat seedlings (Triticum aestivum L.). Frontiers in Plant Science, 9, 597. https://doi.org/10.3389/fpls.2018.00597 [Google Scholar] [PubMed] [CrossRef]
107. Farooq, M. U., Zhu, J. (2019). The paradox in accumulation behavior of cadmium and selenium at different planting times in rice. Environmental Science and Pollution Research, 26(22), 22421–22430. https://doi.org/10.1007/s11356-019-05467-6 [Google Scholar] [PubMed] [CrossRef]
108. Shanker, K., Mishra, S., Srivastava, S., Srivastava, R., Dass, S. et al. (1995). Effect of selenite and selenate on plant uptake of cadmium by kidney bean (Phaseolus mungo) with reference to Cd—Se interaction. Chemical Speciation and Bioavailability, 7(3), 97–100. https://doi.org/10.1080/09542299.1995.11083251 [Google Scholar] [CrossRef]
109. Lin, L., Zhou, W., Dai, H., Cao, F., Zhang, G. et al. (2012). Selenium reduces cadmium uptake and mitigates cadmium toxicity in rice. Journal of Hazardous Materials, 235, 343–351. https://doi.org/10.1016/j.jhazmat.2012.08.012 [Google Scholar] [PubMed] [CrossRef]
Cite This Article
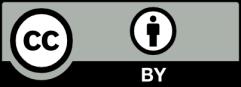