Open Access
ARTICLE
Variation Characteristics of Root Traits of Different Alfalfa Cultivars under Saline-Alkaline Stress and their Relationship with Soil Environmental Factors
1 Northeast Agricultural Research Center of China, Jilin Academy of Agricultural Sciences, Changchun, 130033, China
2 Northeast Institute of Geography and Agroecology, Chinese Academy of Sciences, Changchun, 130102, China
* Corresponding Authors: Fa-Chun Guan. Email: ; Zheng-Wei Liang. Email:
Phyton-International Journal of Experimental Botany 2024, 93(1), 29-43. https://doi.org/10.32604/phyton.2023.046078
Received 18 September 2023; Accepted 15 November 2023; Issue published 26 January 2024
Abstract
Soil salinization is the main factor that threatens the growth and development of plants and limits the increase of yield. It is of great significance to study the key soil environmental factors affecting plant root traits to reveal the adaptation strategies of plants to saline-alkaline-stressed soil environments. In this study, the root biomass, root morphological parameters and root mineral nutrient content of two alfalfa cultivars with different sensitivities to alkaline stress were analyzed with black soil as the control group and the mixed saline-alkaline soil with a ratio of 7:3 between black soil and saline-alkaline soil as the saline-alkaline treatment group. At the same time, the correlation analysis of soil salinity indexes, soil nutrient indexes and the activities of key enzymes involved in soil carbon, nitrogen and phosphorus cycles was carried out. The results showed that compared with the control group, the pH, EC, and urease (URE) of the soil surrounding the roots of two alfalfa cultivars were significantly increased, while soil total nitrogen (TN), total phosphorus (TP), organic carbon (SOC), and α-glucosidase activity (AGC) were significantly decreased under saline-alkaline stress. There was no significant difference in root biomass and root morphological parameters of saline-alkaline tolerant cultivar GN under saline-alkaline stress. The number of root tips (RT), root surface area (RS) and root volume (RV) of AG were reduced by 61.16%, 44.54%, and 45.31%, respectively, compared with control group. The ratios of K+/Na+, Ca2+/Na+ and Mg2+/Na+ of GN were significantly higher than those of AG (p < 0.05). The root fresh weight (RFW) and dry weight (RDW), root length (RL), RV and RT of alfalfa were positively regulated by soil SOC and TN, but negatively regulated by soil pH, EC, and URE (p < 0.01). Root Ca2+/Na+ ratio was significantly positively correlated with soil TN, TP and SOC (p < 0.01). The absorption of Mg and Ca ions in roots is significantly negatively regulated by soil β-glucosidase activity (BGC) and acid phosphatase activity (APC) (p < 0.05). This study improved knowledge of the relationship between root traits and soil environmental factors and offered a theoretical framework for elucidating how plant roots adapt to saline-alkaline stressed soil environments.Keywords
According to statistics, a total of 955 × 106 hm2 of land worldwide is subjected to salt/alkaline stress [1]. The saline-alkaline land area in China is up to 953 million hm2, which has become the main obstacle factor restricting crop yield and economic development [2]. Saline-alkaline land management is currently shifting from saline-alkaline land-adapted to crop to crop-adapted to saline-alkaline land, and one of the most promising future approaches is biological management of saline-alkaline land by planting saline-alkaline tolerant plant types [3]. Therefore, the study of plant adaptation mechanism to saline-alkaline stress is the premise of selecting saline-alkaline tolerant plant cultivars.
Roots are the main organs for plants to absorb water and nutrients, and root traits include root growth, morphology, and physiological traits, which determine plants’ access to underground resources, affect plant growth, survival, distribution, and response to soil environment changes, and play an important driving role in the material circulation and energy flow process of ecosystems [4–7]. Plants control root morphology in some ways to adapt to changes in environmental conditions, strengthen the relationship between them and microorganisms, and maximize nutrient uptake. Plant roots exhibit plasticity in response to environmental changes [8–10]. However, investigations on the variety of root attributes have been limited by the challenge of root acquisition and the intricacy of root structure.
Soil environmental factors, including soil salinity, soil nutrients, and soil enzyme activities, are the main factors affecting plant growth and development. Environmental factors in the soil give plant roots feedback and cause corresponding root modifications. Plants will increase root length to adapt to drought environments [11], and under high pH stress, plants will reduce morphological parameters such as root length, root surface area, and root volume [12]. When soil phosphorus is deficient, plants will show inhibition of taproot length, increase of lateral roots and root hairs, and shallow root distribution [13]. Soil enzymes are mainly derived from the secretions of microorganisms and plant roots and the enzymes released by plant and animal residues, which are catalysts for soil biochemical reactions. At the same time, they also participate in the important processes of carbon, nitrogen, and phosphorus turnover and energy conversion in soil [14]. Soil enzyme activity is related to salt stress intensity, and with the increase of salt concentration, soil urease activity significantly decreases [15]. However, soil urease can be promoted by low-concentration salt stress [16]. Soil phosphatase activity can be induced by low available phosphorus levels [17]. Plant nutrient bioavailability is significantly influenced by soil enzyme activities, which in turn impacts plant growth rates [18,19]. Therefore, it is crucial to understand how plant root characteristics interact with soil environmental factors in order to understand how plant roots adapt to the saline-alkaline-stressed soil environment.
Alfalfa (Medicago sativa L.) is a high-yield and good-quality forage with high salt tolerance [20]. Planting alfalfa can reduce soil salinity and pH value, and rhizobium in its root system can fix nitrogen and improve the soil environment [21]. Our previous research was based on the indoor addition of an alkaline solution to simulate a saline-alkaline stress environment and explained that different alfalfa cultivars can alleviate salt-alkaline stress damage by adjusting their physiology, biochemistry, and gene expression, and saline-alkaline tolerant cultivars are better adapted to saline-alkaline stress environment than saline-alkaline sensitive cultivars [22]. However, investigations on the traits of different alfalfa cultivars such as root biomass, root morphological parameter change, and amount of mineral nutrient accumulation in the roots under naturally saline-alkaline soil circumstances, are still lacking.
Therefore, this study took alfalfa cultivars with different saline-alkaline tolerance as the research object. The differences in root traits of alfalfa cultivars with different saline-alkaline tolerance under saline-alkaline stress were analyzed by measuring root growth, root morphology parameters, and root ion contents. At the same time, plant root traits and soil properties were explored with correlation analysis of the soil salinity indexes, soil nutrient indexes, and soil activities of key enzymes involved in soil carbon, nitrogen, and phosphorus cycles. The research results can provide a theoretical basis for revealing the adaptation mechanism of plant roots to saline-alkaline stressed soil environments. Meanwhile, it provides an important reference value for breeding saline-tolerant cultivars in saline-alkali land restoration.
The experimental base of Jilin Academy of Agricultural Sciences is located in Nanguan District, Changchun City, Jilin Province, at 125°33′ East longitude, and 43°89′ north latitude. The region has the characteristics of a mid-temperate continental monsoon climate. The average monthly temperature varies between 15°C–28°C and the monthly precipitation varies between 15.2–23.6 mm during the experiment period.
The experiment was done on July 05, 2022, in the glass greenhouse of the Jilin Academy of Agricultural Sciences. The test soil was derived from the black soil of the Jilin Academy of Agricultural Sciences and soda saline-alkaline soil from the National Da’an Alkaline Soil Test Station. The black soil was the non-saline-alkaline soil (Control) group (pH 7.71 ± 0.06; EC, 120.00 ± 2.87), and the mixed saline-alkaline soil was created by combining black soil and saline-alkaline soil at a ratio of 7:3, and it was designated as the saline-alkaline stress treatment group (Saline-alkaline stress, pH, 8.51 ± 0.02; EC 327.33 ± 4.67). An alkaline-tolerant cultivar, Gongnong NO.1 (GN), and an alkaline-sensitive cultivar, Algonquin (AG), were selected based on our previous study [22]. Each bucket was filled with about 5 kg of soil, and each bucket was seeded in the five-point method of acupoint sowing. Seeds were disinfected with 6% sodium hypochlorite, then 10 seeds were planted at each acupoint, and the seedlings were thinned after emergence to ensure that there were 5 seedlings remaining in each bucket and each hole with the same growth, that is, 15 seedlings in each bucket. All of the experiments were conducted with three biological replicates, each consisting of 3 plastic black buckets (height 21.0 cm, diameter 20.7 cm) of alfalfa seedlings, with 15 seedlings in each bucket. After sowing, irrigation was done with tap water (EC 1.05 mS/cm, pH 7.52), and post-emergence field management was not implemented with fertilization except manual weeding when necessary.
2.3 Measurement Items and Methods
We selected 7 weeks as the sampling time based on the phenotypic characteristics of leaf yellowing in alkaline-sensitive cultivar AG. Five plants were carefully excavated from each treatment, and the soil surrounding the roots of alfalfa was collected by shaking the root method [23], air-dried at room temperature, homogenized, and screened by 2 mm for the determination of soil chemical properties and soil enzyme activities.
The root samples were rinsed with deionized water and dried with absorbent paper. Then, the roots of these five seedlings were scanned with an Epson Expression 10000XL (Epson America Inc., Long Beach, CA, United States) root scanner using the method described by Zhang et al. [12]. Then the WinRHIZO (Regent Instruments Canada Inc., Ville de Québec, QC, Canada) root analysis software was used to analyze root morphological parameters root length (RL), root surface area (RS), root tips (RT), and root volume (RV). Then, five seedlings under each treatment were collected as a group, the seedlings were separated from the root, the fresh weight of the shoots and roots were determined, then the fresh samples of the seedlings were placed in the oven, green were killed at 105°C for 40 min, and then dried at 75°C to constant weight, and the dry weight of the shoots and roots were weighed, respectively. The ratios of K+/Na+, Ca2+/Na+, and Mg2+/Na+ indexes in the alfalfa roots were measured by the method of Wei et al. [24].
Soil pH and electric conductivity (EC) were determined by potential method (water:soil = 5:1) (DDS-307A, Shanghai Lei-Ci), and soil total nitrogen, phosphorus, potassium (TN, TP, TK), and soil available N, P, K nutrients (AN, AP, AK) were sent to the official laboratory for testing (Testing Department, Northeast Institute of Geography and Agroecology, Chinese Academy of Sciences, China). TN, TP, and AP were determined by a continuous flow analyzer. AN was determined by titration. TK and AK were determined by ICP-AES using inductively coupled plasma emission spectrometry. Soil organic carbon (SOC) was determined by external heating and potassium dichromate titration. Soil α-glucosidase activity (AGC), β-glucosidase activity (BGC), urease activity (URE), alkaline phosphatase activity (APC) were sent to Beijing First Detection Technology Co., Ltd. (China), for determination, and the analysis was determined by spectrophotometer.
2.4 Data Processing and Analysis Methods
Microsoft Excel 2016 software was used to organize the test data, and Origin 9.1 software was used for data plotting. SPSS 20.0 software was used to conduct one-way ANOVA and Duncan’s method for multiple comparisons (α = 0.05). Principal coordinate analysis (PCoA) was carried out using the R “vegan” package (v2.5–7) to identify variations in root biomass and root morphological parameters in different alfalfa cultivars under saline-alkaline stress. The Spearman correlation coefficient in the R ggcor package (v.0.9.8.1) was used to evaluate correlation heatmaps between root traits and soil properties. All data are expressed as the mean ± standard error of the measurements for 3 biological replicates.
3.1 Variation Characteristics of Soil Properties Surrounding Roots of Different Alfalfa Cultivars under Control and Saline-Alkaline Stress Treatment
The soil EC surrounding the roots of GN was 1.91 times that of the control under salt and alkaline stress, whereas the soil EC surrounding the root of AG was 1.96 times that of the control group (Table 1). The soil AN content in GN under saline-alkaline stress treatment increased by 88.46% compared with that of the control group. However, the soil AN content in AG decreased by 25.66% compared with the control group. The soil AN content of GN was significantly higher than that of AG (p < 0.05). Saline-alkaline stress had no significant effects on soil AP, AK, and soil TK in either GN or AG. Compared with the control treatment, soil TN content and soil total phosphorus content of GN and AG under saline-alkaline stress treatment were significantly decreased, but there was no significant difference among cultivars (p > 0.05). The SOC of saline-alkaline-induced GN and AG decreased by 2.20% and 17.49%, respectively, compared with the control group.
Under saline-alkaline stress, the LAP of soil surrounding GN roots decreased by 9.69% compared with the control, while that of AG soil increased by 60.60% compared with the control group (Table 2). The URE of soil surrounding GN roots under salt-alkaline stress was increased by 72.38% compared with the control group. However, the URE of soil surrounding AG roots increased by 33.88% compared with the control group. The AGC of GN soil was decreased by 55.95% compared with the control group, while the AGC of soil surrounding AG roots was decreased by 51.91% compared with the control group. The BGC of soil surrounding GN roots was not significantly different from that of the control group under saline-alkaline treatment, but the BGC of soil surrounding AG roots was significantly lower than that of the control group, which decreased by 19.43%. The APC of soil surrounding GN roots was increased by 11.44%, while that of AG was decreased by 9.92%.
3.2 Effects of Saline-Alkaline Stress on Plant Biomass Accumulation of Different Alfalfa Cultivars
After 7 weeks of saline-alkaline stress, the leaf SPAD value of GN was not significantly changed compared with the control group, while the leaf SPAD value of AG was significantly decreased (p < 0.05) (Fig. S1). Saline-alkaline stress significantly affected root dry weight per plant and root fresh weight per plant (p < 0.01), which were 71.81% and 50.44% lower than the control group, respectively. Under saline-alkaline stress, root dry weight per plant and root fresh weight per plant were significantly different between the two alfalfa cultivars (p < 0.01), the root dry weight and fresh root weight per plant of GN were 3.13 times and 1.76 times AG, respectively (Figs. 1A and 1B). However, the shoot biomass of the two cultivars was not significantly different under different treatments (Figs. 1C and 1D).
Figure 1: Changes of root dry weight (A), root fresh weight (B), shoot dry weight (C) and shoot fresh weight (D) in different alfalfa cultivars under saline-alkaline stress. Values are the mean ± standard error, n = 3. NS and Asterisks represent no significant difference or a significant difference between cultivars or between treatments (**p < 0.01) based on Student’s t-test, respectively
3.3 Effects of Saline-Alkaline Stress on Root Morphological Parameters of Different Alfalfa Cultivars
As can be seen from Fig. 2, the number of root tips, root surface area, and root volume of alkaline-sensitive cultivar AG under saline-alkaline stress were significantly reduced compared with the control group, by 61.16%, 44.54%, and 45.31%, respectively. There was no significant difference in root growth parameters of saline-alkali tolerant variety GN under saline and alkaline stress. Under the same stress conditions, plant cultivars have different tolerance to saline-alkaline stress because of their different tolerance to salt and alkaline stress. Under Control treatment, there was no significant difference in root morphological parameters between GN and AG, but under saline-alkaline stress, there were significant differences in root length, number of root tips, root surface area, and root volume between GN and AG, indicating that saline-alkaline stress had more serious effects on root morphological parameters of saline-alkali sensitive cultivars.
Figure 2: Photographs of alfalfa seedling root morphology were taken after alkaline treatment for 7 weeks (A). Bar = 5 cm. Changes of root length (B), root surface area (C), root tips (D), and root volume (E) in different alfalfa cultivars under saline-alkaline stress. GN and AG represent the alkaline-tolerant cultivar, Gongnong NO.1 (GN), and the alkaline-sensitive cultivar, Algonquin (AG), respectively. NS and Asterisks represent no significant difference or a significant difference between cultivars or between treatments (*p < 0.05, ** p < 0.01) based on Student’s t-test, respectively
3.4 Effects of Saline-Alkaline Stress on Mineral Nutrient Uptake in Roots of Different Alfalfa Cultivars
As can be seen from Table 3, the accumulation of Na+ in the roots of GN was lower than that of AG under saline-alkaline stress. Under saline-alkaline stress, the content of Na+ in the root system of GN was 2.26 times that of GN and 4.42 times that of AG. The K+/Na+ ratio of saline-alkaline stress-induced GN was decreased by 54.93% compared with the control group, and the K+/Na+ ratio of saline-alkaline stress-induced AG was decreased by 72.86%. The Ca2+/Na+ ratio of saline-alkaline stress-induced GN was reduced by 71.33% compared with the control group, and the Ca2+/Na+ ratio of alkali stress-induced AG was reduced by 77.76%. In addition, the Na+, K+/Na+, and Ca2+/Na+ ratios of GN were significantly higher than those of AG under the same saline-alkaline stress.
3.5 Interaction between Alfalfa Root Traits and Soil Environmental Factors under Saline-Alkaline Stress
The root morphological parameters of two alfalfa cultivars under different saline-alkaline stress were shown by principal component analysis. The contribution rate of Dim1 is 93.5% and that of Dim1 is 6.5% (Fig. 3). The distribution of GN in the control group and the saline-alkaline group was concentrated in the upper axis, while the distribution of AG in the control group was concentrated in the lower axis, and the distribution of AG in the salt-alkali treatment group was concentrated in the upper axis, indicating that the growth response of the two alfalfa cultivars to saline-alkaline stress was obvious.
Figure 3: Principal coordinate analysis (PCoA) based on root biomass and root morphological parameters in different alfalfa cultivars under saline-alkaline stress. Control-GN, Control-AG, SA-GN, and SA-AG represent the alfalfa cultivar GN or AG in the non-saline-alkaline-stress (control) group or the saline-alkaline-stress group, respectively
Spearman correlation analysis showed that the root traits of the two cultivars were correlated with soil environmental factors (Fig. 4, Table S1). The FRW, FDW, RL, RV, and RS were significantly positively correlated with soil TN (p < 0.01), RFW, RL, RS, and RT were significantly positively correlated with SOC (p < 0.05), indicating that the root traits of alfalfa were mainly positively regulated by soil TN and SOC. However, the FRW, RFW, RL, RV, and RS were negatively correlated with soil URE activity (p < 0.01), root biomass and RT were negatively correlated with soil pH (p < 0.01), and the soil EC value was significantly negatively correlated (p < 0.05), indicating that the root traits of alfalfa were mainly negatively regulated by soil URE, soil pH and EC.
Figure 4: Heatmaps of Spearman correlation coefficients between root traits and soil environmental factors in different alfalfa cultivars under saline-alkaline stress. The shading from blue to red represents a low-to-high positive correlation. *p < 0.05, **p < 0.01. RFW, RDW, RL, RS, RV, RT, pH, EC, AN, AP, AK, TN, TP, TK, SOC, LAP, URE. AGC, BGC, and ACP represent root fresh weight, root dry weight, root length, root surf area, root volume, root tips, soil pH, soil electrical conductivity, soil available nitrogen content, soil available phosphorus content, soil available potassium content, soil total nitrogen content, soil total phosphorus content, soil total potassium content, soil organic carbon content, soil leucine aminopeptidase activity, soil urease activity, soil α-glucosidase activity, soil β-glucosidase activity and soil acid phosphatase activity, respectively
The root nutrient uptake was also affected by soil environmental factors. There was a significant positive correlation between root Na+ content and soil pH and an extremely significant positive correlation with soil EC. However, the root K+/Na+ ratio and Mg2+/Na+ ratio were negatively correlated with soil pH (p < 0.05), was significantly negatively correlated with soil EC (p < 0.01), the root Ca2+/Na+ ratio was significantly negatively correlated with soil pH (p < 0.01), and the soil EC was significantly negatively correlated (p < 0.05). The root Ca2+/Na+ ratio was positively correlated with soil TN, TP, and SOC (p < 0.01). The root K+/Na+ ratio and Mg2+/Na+ ratio were significantly positively correlated with soil TN, and soil AGC (p < 0.01), and were significantly positively correlated with TP (p < 0.05). The root Ca2+, Ca2+/Na+ ratio, K+/Na+ ratio, and Mg2+/Na+ ratios were negatively correlated with soil URE activity (p < 0.01), and root Ca2+ and Mg2+ were negatively correlated with soil BGC and APC activities.
4.1 Different Alfalfa Cultivars’ Root Traits Respond Differently to Saline-Alkaline Stress
Roots are an important interface between plants and the soil environment. As a network channel for the material, energy, and information cycles of plants, the changes in root structure to different soil environments represent the adaptation strategies and evolutionary directions of plants [7]. The most direct adaptation methods for the efficient absorption and use of soil nutrients under challenging conditions include changes in root shape, development, and physiological features [25,26]. Soil salinity strongly affects the horizontal and vertical distribution of plants, as well as root length and size [11]. In this study, compared with the control group, there were no significant differences in root biomass, RL, RT, and RS of GN under saline-alkaline stress. However, root growth and morphological parameters of AG were significantly reduced. The root growth of the saline-alkali sensitive variety AG was significantly inhibited by the saline-alkaline stress of this intensity, and the root growth of the saline-alkali tolerant variety GN was significantly higher than those of the sensitive variety under the same saline-alkaline stress condition (Figs. 1 and 2). The above results indicate that under specific salt-alkaline stress intensity, GN can enhance its adaptability to salt-alkaline stress by adjusting its root configuration [26]. Under environmental stress, the root system can enhance its competitiveness for resources by changing root morphology. RL and RS determine the ability of roots to absorb water and nutrients [27]. In addition, under the same saline-alkali stress condition, the Na+, the ratios of K+/Na+, Ca2+/Na+, and Mg2+/Na+ of GN root was significantly higher than that of AG, indicating that the absorption of Na+ by GN root under saline-alkaline stress was reduced. This result is consistent with the above-ground ion accumulation data of various alfalfa cultivars in our previous studies [24], and it also confirms that GN has stronger adaptability to salt-alkali stress by maintaining a high K+/Na+ ratio, Ca2+/Na+ ratio, and Mg2+/Na+ ratio to adapt to salt-alkaline stress environment.
4.2 Relationship between Root Traits of Different Alfalfa Cultivars and Soil Environmental Factors under Saline-Alkaline Stress
In this study, PCA principal component analysis data showed that the response mechanism of two alfalfa cultivars to saline-alkaline stress was different, and the growth of AG cultivars was significantly different (Fig. 3). Spearman heatmap analysis showed that the root biomass and root morphological parameters of two alfalfa cultivars were significantly positively correlated with soil SOC and TN (p < 0.05), indicating that the root traits of the two cultivars were significantly positively regulated by these factors, which was consistent with the research results of Luo et al. [28], confirming that root activity may be one of the important factors affecting soil C and N accumulation. However, root biomass and root morphological parameters were negatively correlated with soil pH and soil EC (p < 0.05), indicating that the root traits of alfalfa were mainly negatively regulated by soil pH and soil EC. The result was consistent with the study of González-Orenga et al. [11], indicating that the higher the soil EC value, the higher the soil salt content, resulting in osmotic stress and Na+ toxicity to roots, thus inhibiting plant growth.
In the results of this study, RFW and RDW, RL, RS, RV and RT of two alfalfa cultivars were significantly negatively correlated with soil URE (p < 0.01) (Fig. 4), indicating that the limited available nitrogen content in soil will promote the secretion of more extracellular enzymes by microorganisms, thus accelerating the decomposition of soil organic matter and generating a positive rhizosphere excitation effect to accelerate the process of soil nitrogen transformation and thus improve crop nitrogen nutrition [29]. In addition, the number of root tips was positively correlated with soil AGC (p < 0.05), which is consistent with the results of Yang et al. [30], indicating that soil salinity has a significant impact on soil carbon metabolism, possibly because soil salinity can cause a decrease in carbohydrate metabolism and gene abundance of glycosyl-transferase and glycoside hydrolase. We hypothesized that saline-alkaline stress limited the utilization of carbohydrate and amino acid carbon sources, and ultimately affected root growth, but this part of the results needs further study.
The uptake of mineral nutrients by roots is related to the migration of soil nutrients to the surface. There are three main ways for soil nutrients to migrate to the root surface: active interception, mass flow, and diffusion. However, the nutrients obtained by active interception only account for a very small part, and the nutrients obtained by roots are mainly obtained by mass flow or diffusion. Plant roots absorb Ca and Mg elements through the mass flow path, and root mass flow depends on root water absorption and plant transpiration. In this process, a “pull” that continuously pulls water upward is formed in the plant body, resulting in a pressure difference between the soil solution and root surface, which promotes the migration of soil nutrients along with water to the root surface and is ultimately conducive to nutrient absorption [31]. In this study, the Ca2+/Na+ ratio and Mg2+/Na+ ratio of roots were significantly positively correlated with soil total nitrogen content (p < 0.01), Mg2+/Na+ ratio of the root was positively correlated with soil total phosphorus content (p < 0.05), Ca2+/Na+ ratio was significantly positively correlated with soil total phosphorus content (p < 0.01) (Fig. 4), indicating that the higher the soil nitrogen and phosphorus nutrient levels, the more vigorous the plant growth, the stronger the transpiration, and the stronger the relative absorption capacity of Ca and Mg elements of alfalfa. In addition, the Na ion content of root mineral nutrients was significantly positively correlated with soil pH and soil EC (p < 0.05), but root K+/Na+ ratio, Ca2+/Na+ ratio, and Mg2+/Na+ ratio were significantly negatively correlated with soil pH value, and soil EC value (p < 0.05), indicating that an environment with high soil pH and EC values will lead to precipitation of soil Ca2+ and Mg2+ (CaCO3 and MgCO3), leaving fewer ions available for plant absorption [32]. Similarly, a high pH environment outside the roots causes the plant to absorb more Na+, creating an absorption competition with other nutrient cations, including Ca2+ and Mg2+. The more severe the saline-alkaline stress, alfalfa adsorbed too many Na ions, thus inhibiting the absorption of K, Ca, and Mg ions by plants [33]. Mg is an important component of chlorophyll, and P is a component of the cell membrane and ATP. Water soluble C in soil is the internal organization and energy source of plants [34]. Interestingly, in this study, root Mg ion content was significantly negatively correlated with soil BGC and AGC (p < 0.01). We hypothesize that the reason is high Na and high pH under saline-alkaline stress inhibited the absorption of Mg by plants, and plant rhizosphere acidified and secreted low molecular weight organic anions (phenolic com-pounds, organic acids, etc.) to accelerate the decomposition of soil organic carbon [35]. Meanwhile, phosphatase was secreted by roots to mobilize the phosphorus absorption of soil [36] and activate the activities of soil C key enzyme BGC and soil P key enzyme AGC for promoting normal photosynthesis of plants.
The saline-alkaline stress was accompanied by high Na and high pH, which resulted in significant changes in the root morphology of two alfalfa cultivars with different sensitivities to alkaline stress. We speculate that the reasons are as follows: (1) soil structural factors: the saline-alkaline soil often exhibits unique structural problems as a result of certain physical processes (slaking, swelling, and dispersion of clay) and specific conditions (i.e., surface crusting and hard setting) due to the excess sodium (Na+) levels, these problems will affect the movement of water and air in the soil, and ultimately affect the distribution of root morphology [37]; (2) physiological and molecular adaptation of plants: the root activity decreased at high pH levels, which impaired membrane permeability and the ion transfer system, leading to an imbalance between Na+ and K+, and in turn to excessive accumulation of reactive oxygen species (ROS) and decreased the growth of plant root [38]; the alkaline salt causes an increase in Na accumulation as well as a decrease in nutrient content, disturbs the flux of multiple ions in the roots [39]; saline-alkali stress-induced changes in the expression levels of genes involved in signal transduction, transcription factor regulation and saline-alkaline tolerance response in plants, which affected the growth and development of plant roots [40].
In summary, different alfalfa cultivars have different adaptation strategies to saline-alkaline stress. The root biomass and root morphological parameters of saline-alkaline tolerant alfalfa cultivars remain relatively stable, while the saline-alkaline sensitive cultivars respond to saline-alkaline stress by reducing root biomass and adjusting root morphological parameters. Root biomass and root morphological parameters were significantly positively regulated by soil SOC and TN surrounding alfalfa roots, but significantly negatively regulated by soil pH, soil EC, and soil URE. The absorption of Mg and Ca ions in roots is significantly negatively regulated by soil BGC and APC. The above results indicate that alfalfa can change root biomass, and adjust root structure and root ion distribution to adapt to a saline-alkaline stressed soil environment, which is also an adaptive mechanism for alfalfa to survive long-term under saline-alkaline stress environment.
Acknowledgement: Not applicable.
Funding Statement: This research was funded by the Agricultural Science and Technology Innovation Project of Jilin Province (Postdoctoral Fund Project) (CXGC2021RCB007); Agricultural Science and Technology Innovation Project of Jilin Province (Introduction of Doctor and High-Level Talents Project) (CXGC2022RCG008); Jilin Province Science and Technology Development Project (20200403014SF); Agricultural Science and Technology Innovation Project of Jilin Province (CXGC2021ZY036).
Author Contributions: The authors confirm contribution to the paper as follows: conceptualization: Fa-Chun Guan and Zheng-Wei Liang; validation: Fa-Chun Guan and Zheng-Wei Liang; investigation: Tian-Jiao Wei, Guang Li, Yan-Ru Cui, Jiao Xie, Xing-Ai Gao, Xing Teng, and Xin-Ying Zhao; writing—original draft: Tian-Jiao Wei; writing-review and editing: Fa-Chun Guan and Zheng-Wei Liang. All authors reviewed the results and approved the final version of the manuscript.
Availability of Data and Materials: Not applicable.
Ethics Approval: Not applicable.
Conflicts of Interest: The authors declare that they have no conflicts of interest to report regarding the present study.
References
1. Pandey V, Singh K, Singh B, Singh R. New approaches to enhance eco-restoration efficiency of degraded sodic lands: critical research needs and future prospects. Ecol Restor. 2011;29(4):322–5. [Google Scholar]
2. Yang F, An F, Ma H, Wang Z, Zhou X, Liu Z. Variations on soil salinity and sodicity and its driving factors analysis under microtopography in different hydrological conditions. Water. 2016;8(6):227. [Google Scholar]
3. Xia J, Ren J, Zhang S, Wang Y, Fang Y. Forest and grass composite patterns improve the soil quality in the coastal saline-alkali land of the Yellow River Delta, China. Geoderma. 2019;349(20):25–35. [Google Scholar]
4. Tomlinson KW, Sterck FJ, Bongers F, da Silva DA, Barbosa ERM, Ward D, et al. Biomass partitioning and root morphology of savanna trees across a water gradient. J Ecol. 2012;100(5):1113–21. [Google Scholar]
5. Bardgett RD, Mommer L, de Vries FT. Going underground: root traits as drivers of ecosystem processes. Trends Ecol Evol. 2014;29(12):692–9. [Google Scholar] [PubMed]
6. Zhou M, Bai W, Li Q, Guo Y, Zhang WH. Root anatomical traits determined leaf-level physiology and responses to precipitation change of herbaceous species in a temperate steppe. New Phytol. 2021;229(3):1481–91. [Google Scholar] [PubMed]
7. Karlova R, Boer D, Hayes S, Testerink C. Root plasticity under abiotic stress. Plant Physiol. 2021;187(3):1057–70. [Google Scholar] [PubMed]
8. Mai W, Xue X, Feng G, Yang R, Tian C. Can optimization of phosphorus input lead to high productivity and high phosphorus use efficiency of cotton through maximization of root/mycorrhizal efficiency in phosphorus acquisition?. Field Crop Res. 2018;216(1):100–8. [Google Scholar]
9. Xu LL, Wang H, Gao PP, Wu SQ, Zhang CL. Effects of environmental stress on plant root morphology. J Anhui Agric Sci. 2020;48(14):16–9 (In Chinese). [Google Scholar]
10. Shelden MC, Munns R. Crop root system plasticity for improved yields in saline soils. Front Plant Sci. 2023;14:3291. [Google Scholar]
11. González-Orenga S, Llinares JV, Al Hassan M, Fita A, Collado F, Lisón P, et al. Physiological and morphological characterisation of Limonium species in their natural habitats: insights into their abiotic stress responses. Plant Soil. 2020;449(1):267–84. [Google Scholar]
12. Zhang H, Liu XL, Zhang RX, Yuan HY, Wang MM, Yang HY, et al. Root damage under alkaline stress is associated with reactive oxygen species accumulation in rice (Oryza sativa L.). Front Plant Sci. 2017;8(1580). [Google Scholar]
13. Yan XL, Lin ZY, Hu WJ, Ma XQ, L.P. Q. Root morphological characteristics of cunninghamia lanceolata and its foraging strategies. World Forestry Res. 2022;35(1):26–31 (In Chinese). [Google Scholar]
14. Song X, Zhang KK, Huang CC, Huang SM, Yue K, Zhang ZB, et al. Analysis of root characteristics and rhizosphere soil enzyme activities of wheat varieties with different nitrogen efficiencies. J Nucl Agric Sci. 2023;37(3):617–25 (In Chinese). [Google Scholar]
15. Yang D, Tang L, Cui Y, Chen J, Liu L, Guo C, et al. Saline-alkali stress reduces soil bacterial community diversity and soil enzyme activities. Ecotoxicology. 2022;31(9):1356–68. [Google Scholar] [PubMed]
16. Yan SJ, Liu YM, Sun YL, Lin XS, Luo HL, Lin H, et al. Analysis of microbial diversities and enzyme activities of rhizosphere soil of Pennisetum giganteum (giant juncao) under different degrees of saline-alkali stress. Guihaia. 2022;42(3):491–500 (In Chinese). [Google Scholar]
17. Zhang AM. Characteristics of soil phosphorus components and phosphatase activity under different land use patterns (Ph.D. Thesis). University of Chinese Academy of Sciences: China; 2013. [Google Scholar]
18. Peiris C, Gunatilake SR, Wewalwela JJ, Vithanage M. Biochar for sustainable agriculture: nutrient dynamics, soil enzymes, and crop growth. Elsevier; 2019. [Google Scholar]
19. Burns RG, DeForest JL, Marxsen J, Sinsabaugh RL, Stromberger ME, Wallenstein MD, et al. Soil enzymes in a changing environment: current knowledge and future directions. Soil Biol Biochem. 2013;58:216–34. [Google Scholar]
20. Scasta J, Trostle C, Foster M. Evaluating alfalfa (Medicago sativa L.) cultivars for salt tolerance using laboratory, greenhouse and field methods. J Agri Sci. 2012;4(6):90–103. [Google Scholar]
21. Abiala MA, Abdelrahman M, Burritt D, Tran LSP. Salt stress tolerance mechanisms and potential applications of legumes for sustainable reclamation of salt-degraded soils. Land Degrad Devel. 2018;29(10):3812–22. [Google Scholar]
22. Wei TJ, Li G, Wang MM, Jin YY, Zhang GH, Liu M, et al. Physiological and transcriptomic analyses reveal novel insights into the cultivar-specific response to alkaline stress in alfalfa (Medicago sativa L.). Ecotox Environ Safe. 2021;228:113017. [Google Scholar]
23. Zhang Y, Xu J, Riera N, Jin T, Li J, Wang N, et al. Huanglongbing impairs the rhizosphere-to-rhizoplane enrichment process of the citrus root-associated microbiome. Microbiome. 2017;5(1):97. [Google Scholar] [PubMed]
24. Wei TJ, Jiang CJ, Jin YY, Zhang GH, Wang MM, Liang ZW. Ca2+/Na+ ratio as a critical marker for field evaluation of saline-alkaline tolerance in alfalfa (Medicago sativa L.). Agronomy. 2020;10:191. [Google Scholar]
25. Alharby HF, Colmer TD, Barrett-Lennard EG. Salinization of the soil solution decreases the further accumulation of salt in the root zone of the halophyte Atriplex nummularia Lindl. growing above shallow saline groundwater. Plant Cell Environ. 2018;41(1):99–110. [Google Scholar] [PubMed]
26. Wang S, Zhao Z, Ge S, Peng B, Zhang K, Hu M, et al. Root morphology and rhizosphere characteristics are related to salt tolerance of Suaeda salsa and Beta vulgaris L. Front Plant Sci. 2021;12. [Google Scholar]
27. Bauhus J, Khanna PK, Menden N. Aboveground and belowground interactions in mixed plantations of Eucalyptus globulus and Acacia mearnsii. Can J For Res. 2000;30(12):1886–94. [Google Scholar]
28. Luo YQ, Zhao XY, Wang T, Li YQ. Characteristics of the plant-root system and its relationships with soil organic carton and total nitrogen in a degraded sandy grassland. Cao Ye Xue Bao. 2017;26(8):200–6 (In Chinese). [Google Scholar]
29. Sun Y, Xu XL, Yakov K. Mechanisms of rhizosphere priming effects and their ecological significance. Chin J Plant Ecol. 2014;38(1):62–75 (In Chinese). [Google Scholar]
30. Yang C, Lv D, Jiang S, Lin H, Sun J, Li K, et al. Soil salinity regulation of soil microbial carbon metabolic function in the Yellow River Delta, China. Sci Total Environ. 2021;790:148258. [Google Scholar] [PubMed]
31. Nye PH, Marriott FHC. A theoretical study of the distribution of substances around roots resulting from simultaneous diffusion and mass flow. Plant Soil. 1969;30(3):459–72. [Google Scholar]
32. Rengasamy P. Soil processes affecting crop production in salt-affected soils. Funct Plant Biol. 2010;37(7):613–20. [Google Scholar]
33. Shi D, Zhao KF. Effects of NaCl and Na2CO3 on growth of Puccinellia tenuiflora and on present state of mineral elements in nutrient solution. Cao Ye Xue Bao. 1997;6:51–61 (In Chinese). [Google Scholar]
34. Waraich EA, Ahmad R, Ashraf MY. Role of mineral nutrition in alleviation of drought stress in plants. Aus J Crop Sci. 2011;5(6):764–77. [Google Scholar]
35. Yan S, Yin L, Dijkstra FA, Wang P, Cheng W. Priming effect on soil carbon decomposition by root exudate surrogates: a meta-analysis. Soil Biol Biochem. 2023;178:108955. [Google Scholar]
36. White PJ, Hammond JP. Phosphorus nutrition of terrestrial plants. In: The ecophysiology of plant-phosphorus interactions. Springer; 2008. [Google Scholar]
37. Qadir M, Schubert S. Degradation processes and nutrient constraints in sodic soils. Land Degrad Dev. 2002;13:275–94. [Google Scholar]
38. Wang J, Li Q, Zhang M, Wang Y. The high pH value of alkaline salt destroys the root membrane permeability of Reaumuria trigyna and leads to its serious physiological decline. J Plant Res. 2022;135(6):785–98. [Google Scholar] [PubMed]
39. Hu X, Wang D, Ren S, Feng S, Zhang H, Zhang J, et al. Inhibition of root growth by alkaline salts due to disturbed ion transport and accumulation in Leymus chinensis. Environ Exp Bot. 2022;200:104907. [Google Scholar]
40. Fang S, Hou X, Liang X. Response mechanisms of plants under saline-alkali stress. Front Plant Sci. 2021;12:481. [Google Scholar]
Supplementary Materials
Figure S1: Changes of leaf SPAD value in different alfalfa cultivars under saline-alkaline stress. Values are the mean ± standard error, n = 3. NS and Asterisks represent no significant difference or a significant difference between cultivars or between treatments (*p < 0.05) based on Student’s t-test, respectively
Cite This Article
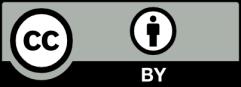