Open Access
ARTICLE
Comprehensive Assessment of the Safety of Eucommia ulmoides Leaf Extract for Consumption as a Traditional Chinese Health Food
1
School of Chemical and Environmental Engineering, Hunan Institute of Technology, Hengyang, 421002, China
2
Guangdong Provincial Key Laboratory of Chemical Measurement and Emergency Test Technology, Guangdong Provincial
Engineering Research Center for Ambient Mass Spectrometry, Institute of Analysis, Guangdong Academy of Sciences (China
National Analytical Center, Guangzhou), Guangzhou, 510070, China
* Corresponding Authors: Jiali Fang. Email: ; Xuesong Wang. Email:
(This article belongs to the Special Issue: Renewable Biomass as a Platform for Preparing Green Chemistry)
Journal of Renewable Materials 2023, 11(7), 3091-3114. https://doi.org/10.32604/jrm.2023.026689
Received 20 September 2022; Accepted 07 November 2022; Issue published 05 June 2023
Abstract
To ensure the export quality of Eucommia ulmoides leaf extract (ELE) and facilitate E. ulmoides leaf inclusion in the directory of traditional Chinese health foods, an overall safety assessment of ELE was performed, including genotoxicity and long-term toxicity, according to the national food safety standards of China. No variations in the reverse mutation number of the nominal bacterial strains were observed under ELE treatment in comparison with the solvent control. Additionally, the micronucleus rates of in vivo mammalian erythrocytes and in vitro mammalian cells under ELE treatment were equivalent to or significantly lower than those of the solvent control. The fold change in the trifluorothymidine resistance mutation frequency of the thymidine kinase gene under ELE treatment was less than three times in comparison with the solvent control, suggesting that ELE did not cause genotoxicity. Moreover, animal experiments showed that the growth performance of rats under ELE treatment was enhanced because the body weights of rats increased. No oxidative injury or inflammatory responses were induced and no histopathological lesions of tissues were detected under ELE treatment. In addition, plasma triglycerides and low-density lipoprotein cholesterol levels significantly decreased, and plasma high-density lipoprotein cholesterol levels significantly increased with ELE treatment, suggesting that ELE was health-promoting. Furthermore, moderate to excellent antimicrobial activities, a favorable anticancer capacity, and superior antioxidant abilities of ELE were found, implying ELE possesses good bioactivities. Therefore, we affirmed ELE is safe to consume as a traditional Chinese health food.Graphic Abstract
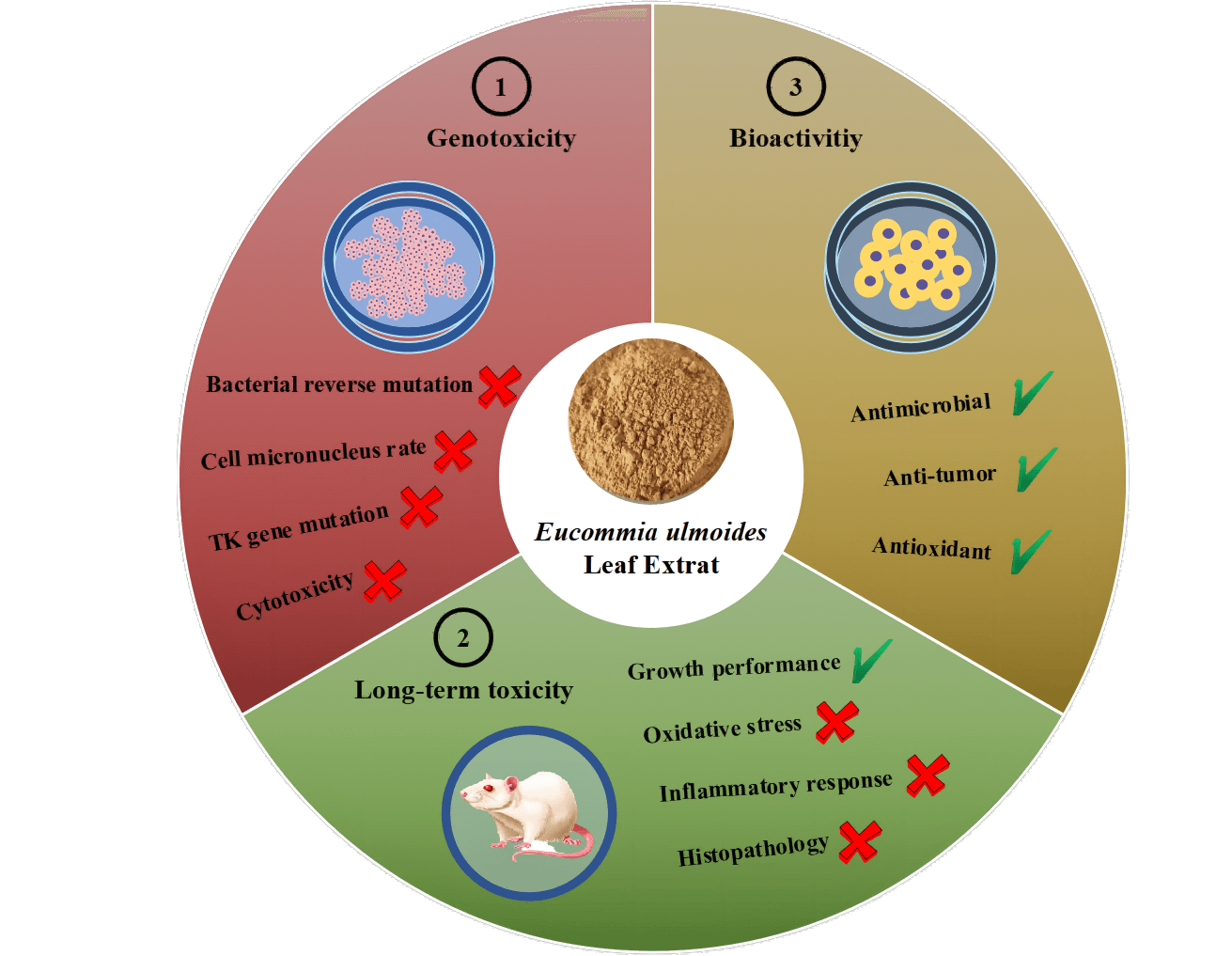
Keywords
Eucommia ulmoides (E. ulmoides) Oliver, a traditional Chinese medicinal plant generally known as “duzhong” in China [1,2], is a monotypic deciduous and dioecious tree and is the only species of the genus Eucommia [3,4]. E. ulmoides originated in China during the glacial movement in the third century and is currently cultivated at an altitude range of 300–1300 m in China, such as in the Hunan, Henan, and Yunnan provinces [5]. E. ulmoides has a long application history, particularly the raw bark. As recorded in the Chinese medical classics Shen Nong Ben Cao Jing and Ben Cao Gang Mu, the raw bark of E. ulmoides has been used as traditional Chinese medicine and has been included in the Chinese Pharmacopoeia [6]. Based on its pharmacological activities, E. ulmoides bark is commonly used as a tonic for organs (liver and kidney) and has been found to prevent miscarriage, regulate blood pressure, and act as an anti-aging agent [7–10]. Although E. ulmoides bark has shown multiple pharmacological effects, the bark is not conducive to large-scale development and utilization due to resource constraints. Therefore, a new renewable resource is urgently needed to compensate for the shortage of bark.
To find an alternative to the bark, an analysis of the chemical components of the bark was necessary. The chemical components of the bark were separated, purified, identified, and found to be lignans, iridoids, flavonoids, phenolics, steroids, terpenoids, and gutta-percha [5]. Of these, lignans and iridoids were identified as the two most important constituents, and are usually denoted as key chemotaxonomic markers. These two constituents were shown to exhibit excellent pharmacological activities, such as the lignans liriodendrin, (+)-pinoresinol di-O-β-D-glucopyranoside, and (+)-syringaresinol, which were found to have a satisfactory antihypertensive effect, and aucubin, genipin, and geniposidic acid of iridoids, which displayed remarkable antihypertension and anti-aging effects. Recently, evidence has shown that the chemical components of the bark are similar to those of the leaves, including lignans, iridoids, phenols, steroids, triterpenes, polysaccharides, and flavonoids [11,12], suggesting that the leaves of E. ulmoides can solve the dilemma of the bark shortage. In addition, numerous studies have reported that E. ulmoides leaves were found to increase bone strength, reduce fattiness, and increase energy metabolism, thus resulting in longevity and improved fertility in humans [13,14]. Moreover, flavonoids of E. ulmoides leaves (e.g., chlorogenic acid (CGA), rutin, ferulic acid, and caffeic acid) displayed good antioxidant activity [15]. Taken together, E. ulmoides leaves are a promising replacement for bark [16]. Furthermore, due to the process of peeling will cause trunk damage (even death) and the bark regeneration speed being slower than leaves, the E. ulmoides leaves may be more suitable for commercialization based on their advantages of picking easily, large amount, and growth every year. Therefore, E. ulmoides leaves are a better renewable material in comparison with the bark, which can meet the ever-increasing demand.
Because the utility and value of E. ulmoides have been widely recognized, it has attracted much attention from abroad and has been successfully traded with other countries, such as the United States, Russia, Britain, and France [17]. In 2019, we formulated the group standards for E. ulmoides leaf extract at the invitation of the China Chamber of Commerce for Import and Export of Pharmaceutical and Health Products, which ensured the export quality of E. ulmoides leaf extract. Furthermore, although the chemical components of the bark are similar to the leaves, some composition differences were determined between them, such as the characteristic component, CGA, which is highly enriched in leaves, whereas its concentration in the bark is below the detection limit. Simultaneously, divergent in other characteristic components composition is observed between leaves and barks, e.g., rutin is only determined in leaves, whereas geniposide and pinoresinol diglucoside are only detected in barks. In addition, apart from the composition difference, the concentrations of the same components (aucubin, geniposidic acid) in leaves also distinct from that of in barks, and their concentrations are significantly higher in barks [18]. Previous studies have demonstrated that CGA induces an antihypertensive effect [19] and significantly reduced the systolic and diastolic blood pressure of hypertensive patients and rats [20,21], and can potentially serve as an antihypertensive drug [22]. Meanwhile, geniposidic acid and aucubin show the abilities of free radical scavenging, anti-inflammatory, and anti-cancer [23]. Therefore, the composition and concentration difference of these characteristic components between the bark and leaves might lead to bioactivity variations, and an overall assessment of the safety of E. ulmoides leaf extract is urgently needed to ensure the continued trade of E. ulmoides leaf extract around the world.
In the present study, genotoxicity tests, cytotoxicity assay, and in vivo long-term animal toxicity studies of E. ulmoides leaf extracts were performed according to the national food safety standards of China. Furthermore, the bioactivity of E. ulmoides leaf extracts was also explored, including analysis of antimicrobial activity, anticancer capacity, and antioxidant abilities. We hypothesized that E. ulmoides leaf extract was safe for serving as a traditional Chinese health food based on its bioactivities. Our study will examine the safety of E. ulmoides extract, which contributes to the application of E. ulmoides leaf extracts.
All of the experiments regarding animals (rats) were approved by the Animal Welfare and Ethics Committee of the Institute of Analysis, Guangdong Academy of Sciences (China National Analytical Center, Guangzhou, China). The permission number is No. 2021002.
The chemical standards (chlorogenic acid, geniposidic acid, aucubin, geniposide, pinoresinol diglucoside, and rutin) were provided by Yuanye Technology Co., Ltd. (Shanghai, China) with a purity ≥99%. Methanol and phosphoric acid were provided by Merck (Darmstadt, Germany), while hexane, petroleum ether, and chloroform were provided by Guangzhou Chemical Reagent Factory (Guangzhou, China). All of the chemical standards and solvents were commercially available and used without dilution unless otherwise specified. A Milli-Q filter system (Millipore, Bedford, MA, USA) was used to prepare ultrapure water. The cells and bacteria trains used in the present study were provided by iPhase (Beijing, China).
2.2 Preparation of E. ulmoides Leaf Extract (ELE)
The dry leaves of E. ulmoides were provided by Hengxin Co., Ltd. (Zhangjiajie, China). After extraction twice with ultrapure water (liquid:solid = 1:10) under the condition of 80°C for 1 h, the extracted liquid was concentrated to 20 Brix under vacuum rotary evaporation. Subsequently, the obtained concentrated liquid was dried by spray drying. During spray drying, the inlet air temperature was 150°C–170°C and, while the outlet air temperature was 90°C–95°C. The extraction yield of ELE is about 25%.
2.3 Composition Analysis of ELE
The chemical composition analysis of ELE was performed on an LC-20A HPLC system (Shimazu, Japan) equipped with a diode array detector. The standard curves of the six chemical standards were plotted first. A Thermo BDS HYPERSIL C-18 analytical column (250 mm× 4.6 mm, 5 μm) was used for target component separation. For separation, the mobile phase solutions consisting of solvent A (0.2% phosphoric acid in water) and solvent B (MeOH) were prepared. A linear gradient elution program was designed as follows: 95% A from 0–17 min; 95%–86% A from 17–45 min; 86%–65% A from 45–55 min; and 65%–55% A from 55–70 min. For analysis, a 10 μL sample was injected into the column, and then the column temperature was maintained at 30°C. The flow rate was set at 1 mL/min. Four characteristic components (geniposidic acid, chlorogenic acid, geniposide, and pinoresinol diglucoside) were detected at 238 nm, while the other two components (aucubin and rutin) were monitored at 208 and 254 nm, respectively. The analysis of physicochemical parameters in ELE (moisture and ash), pesticide residues (hexachlorocyclohexane (HCH), dichlorodiphenyl trichloroethane (DDT), quintozene (PCNB)), microbial content (aerobic bacterial count, coliforms, molds and yeasts, Salmonella, Staphylococcus aureus), and mineral elements were carried out following GB 5009.3-2016, GB 5009.4-2016, GB/T 5009.19-2003, GB/T 5009.136-2003, GB 4789.2-2016, GB 4789.3-2016, GB 4789.15-2016, GB 4789.4-2016, GB 4789.10-2016, and GB 5009.268-2016, respectively.
The bacterial reverse mutation assay, mammalian erythrocyte micronucleus test, in vitro mammalian cell micronucleus test, and in vitro thymidine kinase (TK) gene mutation test on ELE were conducted following GB 15193.4-2014, GB 15193.5-2014, GB 15193.28-2020, and GB 15193.20-2014, respectively. All of these standards were in line with the OECD guidelines with appropriate modification, including OECD 471 bacteria reverse mutation test, OECD 474 mammalian erythrocyte micronucleus test, OECD 487 in vitro micronucleus test, and OECD 490 in vitro mammalian cell gene mutation tests using the thymidine kinase gene.
For bacterial reverse mutation assay, the plate incorporation technique was used. Briefly, five nominal bacterial strains were adopted, including four Salmonella typhimurium strains (TA1535, TA100, TA98, TA97a) and one Escherichia coli strain (WP2uvrA). Four dose solutions of ELE (5, 2.5, 1.25, 0.625 mg/dish) were set with fold changes of two. Pure water was selected as solvent control. 2-aminoanthracene (2-AA), sodium azide (NaN3), 2-aminofluerene (2-AF), methyl methanesulfonate (MMS), and sodium p(dimethylamino) benzenediazo sulfonate (Dexon) were used as the positive control. The culture medium (25 mL/dish) was prepared and stored at 37°C in dark for 24 h. Then, a mixture consisting of agar medium (2 mL), bacteria solution (0.1 mL), ELE solution (0.1 mL), and phosphate buffer (0.5 mL) was added. If the experiment was conducted under metabolic activation conditions, S9 solution (0.5 mL) was added too and denoted as +S9; otherwise, it was defined as −S9. After solidification, the plates were turned upside down and cultivated at 37°C for another 72 h. The reverse mutation number of bacterial strains was recorded.
For the mammalian erythrocyte micronucleus test, three dose solutions of ELE (10000, 5000, 2500 mg/kg) were prepared with a fold changes of two. The 10000 mg/kg was selected as the highest dose based on the rule of 50% lethal dose higher (LD50) than 10 g/kg. Pure water was selected as solvent control. Cyclophosphamide (CTX, 40 mg/kg) was used as the positive control. Ten rats (half male and half female) were used for each dose and ELE solution was given twice in 30 h by the method of gavage. Then, marrow fluids were harvested at 36 h and mixed with fatal bovine serum (FBS) on a slide. After smearing and drying, the slide was fixed with methanol for 5 min and stained with Giemsa for 15 min. Consequently, the slide was washed with phosphate buffer saline (PBS, 1/15 mol/L) and sealed. Two thousand cells were counted and the micronucleus rate (‰) was therefore calculated.
For in vitro mammalian cell micronucleus test, three dose solutions of ELE (5.0, 2.5, 1.25 mg/mL) were prepared with a fold changes of two. The highest dose was set based on the maximum concentration (5.0 mg/mL) without cytotoxicity. Pure water was selected as solvent control. CTX (20 ug/mL) and mytomycin-C (MMC, 0.25 ug/mL) were used as the positive control. If the experiment was conducted under metabolic activation conditions, S9 and CTX solutions were added and denoted as +S9; otherwise, MMC was added and denoted as −S9. Cells with a density of 1 × 105 cells/mL were cultured in a tissue culture flask under the condition of 5% CO2 and 37°C. After removing the medium, ELE (1%) and S9 (10%) solutions were added, together with the complete medium (exclusion FBS). These cells were cultured for 4 h at the same condition and were washed with PBS three times. Consequently, a complete medium was added and cultured for another 24 h. After washing with PBS twice and digestion with 0.25% trypsin solution for 5 min, the cells were centrifuged under 1000 r/min for 5 min. The cells were harvested by discarding supernate and then fixed with stationary liquid (2 mL) three times. After natural drying, the cells were stained with Giemsa for 15 min. Two thousand cells were counted and the micronucleus rate (‰) was therefore calculated.
For in vitro TK gene mutation test, three dose solutions of ELE (5.0, 2.5, 1.25 mg/mL) were prepared with a fold changes of two. The highest dose was set based on the maximum concentration (5.0 mg/mL) without cytotoxicity. Pure water was selected as solvent control. CTX (50 ug/mL) and methyl methanesulfonate (MMS, 5.0 ug/mL) were used as the positive control. If the experiment was conducted under metabolic activation conditions, S9 and CTX solutions were added and denoted as +S9; otherwise, MMs were added and denoted as −S9. Cells with a density of 5 × 105 cells/mL were used and ELE solution (1%) was added. These cells were shaken under 37°C for 3 h and centrifuged to remove supernate. The obtained cells were washed with PBS twice and resuspended by 10% RPMI 1640 medium. The cell density was adjusted to 2 × 105 cells/mL. The plating efficiency at 0 day (PE0) was measured. A cell density of 8 cells/mL (0.2 mL) was inoculated into a 96-well plate and cultured under the condition of 37°C and 5% CO2 for 12 d. For expression, the cell suspension was cultured for 2 d and the cell density was kept below 106 cells/ml. The plating efficiency at the second day (PE2) was determined as the same procedure as PE0. Meanwhile, the trifluorothymidine (TFT) resistance mutation frequency (MF) was detected. The expression cells of the second day were adopted and the cell density was adjusted to 1 × 104 cells/mL. TFT (3 μg/mL) was added and inoculated into a 96-well plate (0.2 mL). The prepared plate was cultured under the condition of 37°C and 5% CO2 for 12 d. The number of wells with mutant colony growth was counted.
The cell viability (%) of CHO, CHL, V79, and human hepatocellular liver carcinoma (HepG2) cells under ELE treatment was determined based on a methyl thiazolyl tetrazolium (MTT) cell proliferation assay. A cell density of 1 × 105/mL was prepared and then 100 μL of the cell suspension was added to the wells of 96-well plates. Medium alone (100 μL) was prepared as a negative control. The prepared plates were incubated at 37°C and 5% CO2 for 12 h. Subsequently, MTT solution (5 mg/mL, 10 μL) was added to each well and incubated for another 6 h. After incubation, the medium of each well was removed and 100 μL of dimethyl sulfoxide (DMSO) was added. The obtained reaction system was mixed thoroughly and the OD value of each well was measured at 490 nm. Three concentrations of ELE (25, 12.5, and 6.25 mg/mL) were used for analyzing the cell viability of CHO, CHL, and V79 cells, while six concentrations of ELE (40.0, 20.0, 10.0, 5.0, 1.0, 0.1 μg/mL) were used for analyzing the cell viability of HepG2 cells. Cisplatin was chosen as the positive drug for evaluating an anticancer effect against HepG2 cells.
2.6 Long-Term Toxicity Testing
2.6.1 90-Day Repeated Dose Toxicity Study with the Following Subsections
Long-term toxicity testing of ELE was conducted following GB15193.13-2015. Sprague-Dawley rats, about 100 g each (n = 80, half male and half female), were randomly assigned to four groups: A group was the basal diet; B, C, and D groups were the basal diet supplemented with 1000, 500, or 200 mg/kg of ELE, respectively. The basal diet was provided by Jiangsu Xietong Shengwu Co., Ltd. (Nanjing, China). All of the rats were allowed free access to water and were fed under constant conditions of a 12 h light and 12 h dark cycle in the China National Analytical Center, Guangzhou (Zhongshan, China). The room temperature (23 ± 1°C) and relative humidity (50% ± 5%) were constant during the entire experimental period of 90 days.
Blood samples of each group were obtained aseptically from the abdominal aortic vein of rats after 90 days of feeding. Then, the blood samples were immediately centrifuged at 3000 r/min for 15 min. All of the blood samples were stored at −80°C before use. Additionally, seven organs (brain, liver, spleen, heart, kidney, adrenal glands, and testis) were collected and weighed individually. Two intestinal tissues (duodenum and ileum) were also collected and stored in 4% paraformaldehyde until further use.
2.6.3 Biochemical and Hematological Analysis
Blood routine indexes (white blood cell (WBC), neutrophil (Neu), lymphocyte (Lym), monocyte (Mon), eosinophil (Eos), basophil (Bas), red blood cell (RBC), hemoglobin (HGB), hematocrit value (HCT), mean corpuscular volume (MCV), mean corpuscular hemoglobin (MCH), mean corpusular hemoglobin concerntration (MCHC), red blood cell distribution width-coefficient of variation (RDW-CV), red blood cell distribution width-standard deviation (RDW-SD), platelet (PLT), mean platelet volume (MPV), platelet distribution width (PDW), thrombocytocrit (PCT)) and blood biochemical indexes (albumin (ALB), alkaliphosphatase (ALP), alanine aminotransferase (ALT), aspartate aminotransferase (AST), creatinine (CREA-S), glucose (Glu-G), total cholesterol (TC), triglyceride (TG), low-density lipoprotein cholesterol (LDL-C), high-density lipoprotein cholesterol (HDL-C), total phosphorus (TP), UREA, γ-glutamyl transpeptidase (γ-GT)) were determined by using relevant detection kits (Mindray, Shenzhen, China). The blood antioxidant indexes (superoxide dismutase (SOD), glutathione (GSH), molondialdehyde (MDA), catalase (CAT)) and inflammatory factors (tumor necrosis factor-α (TNF-α), Interleukin-1β (IL-1β), Interleukin-6 (IL-6)) were also determined by using relevant detection kits from GuangZhou KYDbio Technology Co., Ltd. (Guangzhou, China). All of the analytical procedures were strictly in accordance with the instruction manual of each kit.
2.6.4 Histopathological Examination
Five organs (liver, spleen, kidney, stomach, and pancreas) and two intestinal tissues (duodenum and ileum) were dehydrated with graded alcohol and xylene, and the tissues were embedded in paraffin. After solidification, the obtained paraffin block was cut into 4-μm sections and stained with hematoxylin and eosin. An inverted microscope (ECHO, Chicago, Illinois, USA) was used to observe the tissue morphology. The villus height and the crypt depth of the duodenum and ileum were measured by using an automatic image analyzer (Olympus DP73 camera, Japan). A minimum of six villi and 10 crypts that were well-oriented were obtained from different parts of each intestinal tissue sample.
The antimicrobial activities of ELE against Escherichia coli, Staphylococcus aureus, Pseudomonas aeruginosa, and Aspergillus niger were determined following GB 15979-2002 with minor modifications. Two concentrations of each microbe (1 × 103 and 1 × 104 CFU/mL) and two concentrations of ELE (25.0 and 5.0 mg/L) were prepared. The prepared microbial suspension (100 μL) and ELE solution (0.5 mL) were added to a petri dish with 15 mL Mueller Hinton/potato dextrose agar medium. After solidification, the petri dish was turned over and incubated for 36 h. The incubation temperature of E. coli, S. aureus, and P. aeruginosa was 36°C, while the incubation temperature of A. niger was 15°C. The experiment was repeated three times. Pure DMSO was used as a negative control. The bacterial suspension was used as a blank control. The antimicrobial activity was then calculated.
2.8 Free Radical Scavenging Capacity
Four typical free radicals (hydroxyl radical (OH), 1,1-diphenyl-2-picrylhydrazyl radical (DPPH⋅), 2-phenyl-4,4,5,5-tetramethylimidazoline-1-oxyl 3-oxide radical (PTIO⋅), 2,2’-azino-bis (3-ethylbenzothiazoline-6-sulfonic acid radical (ABTS)) were selected to assess the in vitro free radical scavenging rate of ELE. The detection process of the four free radicals was accorded to the method reported by Li et al. [24].
All data were analyzed by using GraphPad 8.0 (Diego, California, USA) and SPSS 19.0. One-way analysis of variance (ANOVA) and the Tukey test were performed to conduct multiple comparisons. All data were expressed as mean ± standard deviation (SD), with P < 0.05 considered statistically significant.
The composition of ELE is listed in Table 1. The concentrations of crude polysaccharide, total polyphenol, total flavonoid, and protein in ELE were 2.06%, 17.35 g/100 g, 5.27 g/100 g, and 1.62 g/100 g, respectively. Four major characteristic components of ELE (chlorogenic acid, aucubin, geniposidic acid, and rutin) were determined and their concentrations were 81.73, 55.51, 86.55, and 7.55 mg/g, respectively. Based on these active ingredients, ELE likely confers multiple pharmacological activities, such as antiviral, anti-inflammatory, and antioxidant [25–28]. Physiochemical properties, pesticide residues, microbiological components, and mineral element levels in ELE were also measured and all met the group standards for E. ulmoides leaf extract. ELE underwent a safety assessment and bioactivity analysis without findings unless otherwise specified. The chromatographic peaks of standards and ELE are shown in Fig. S1.
3.2 Bacterial Reverse Mutation Assay of ELE
The bacterial reverse mutation assay is a fundamental genetic toxicology test, which is essential in assessing the genotoxic capabilities of food and drugs [29]. In this study, the reverse mutation number of the five nominal bacterial strains (TA1535, WP2uvrA, TA100, TA98, TA97a) under positive control (+/−S9) were either three or two times higher than that under solvent control (Table 2), suggesting that mutagenesis was triggered under positive control. Interestingly, the reverse mutation numbers of the five nominal bacterial strains under ELE treatment were equivalent to that under solvent control, and the fold changes of the reverse mutation numbers between ELE treatment and solvent control were less than two, no matter if the concentration of ELE was low or high, indicating that ELE did not cause mutagenicity.
3.3 Mammalian Erythrocyte Micronucleus Test of ELE
Currently, the in vivo mammalian erythrocyte micronucleus test is the most commonly performed regulatory test in the genotoxicity study [30]. Therefore, the mammalian erythrocyte micronucleus test (Table 3) was carried out on ELE. The micronucleus rates of mammalian erythrocyte under positive control was significantly higher than that under solvent control, indicating that elevated mammalian erythrocyte micronucleus rate was observed under positive control. In contrast to the positive control, the mammalian erythrocyte micronucleus rate under ELE treatment was close to the rate under solvent control, no matter if the concentration of ELE was low or high, implying that ELE did not cause an elevated mammalian erythrocyte micronucleus rate.
3.4 In Vitro Mammalian Cell Micronucleus Test of ELE
Similar to mammalian erythrocyte micronucleus test, the in vitro mammalian cell micronucleus test is one of the tests most often used and required by regulations targeting food safety [31] and the results of in vitro mammalian cells micronucleus test on ELE is listed in Table 4. The micronucleus rates of mammalian cells under positive control (+/−S9) were all significantly higher than those under solvent control, indicating that elevated mammalian cells’ micronucleus rates were determined under positive control. In contrast to the positive control, the micronucleus rates of mammalian cells under ELE treatment were close to or significantly lower than the rates under solvent control, no matter if the concentration of ELE was low or high, suggesting that ELE did not cause an elevated mammalian cells micronucleus rate.
3.5 In Vitro TK Gene Mutation Testing of ELE
To better understand the mechanistic implications regarding in vitro genotoxicity test, the TK gene mutation assay was developed to provide higher specificity toward DNA-damaging reagents [32]. The TFT resistance mutation frequency (T-MF) of the TK gene under positive control (+/−S9) was three times higher than under solvent control (Table 5), indicating that the TK gene mutagenicity was confirmed for the positive control. Although the T-MF of the TK gene under ELE treatment was higher than under solvent control, the fold changes of the T-MF under ELE treatment were less than three times the fold changes of the solvent control, no matter if the concentration of ELE was low or high, indicating that ELE did not cause TK gene mutations.
Evaluation of genotoxicity is a fundamental part of food safety assessment due to the potential health problems caused by genotoxicity. According to the national standards of food safety (GB 15193.4-2014, GB 15193.5-2014, GB 15193.28-2020, and GB 15193.20-2014), four genetic toxicity tests (including the bacterial recovery mutation test, mammalian erythrocyte micronucleus test, in vitro mammalian cells micronucleus test, and in vitro TK gene mutation test) were conducted in the present study. Interestingly, the bacterial reverse mutation assay results showed that the reverse mutation numbers of the tested bacterial strains under ELE treatment were equivalent to that under solvent control, suggesting that ELE does not cause genetic toxicity. Accordingly, the micronucleus rates of mammalian erythrocytes and in vitro mammalian cells under ELE treatment were close to or significantly lower than those of the solvent control, showing ELE did not cause chromosomal aberrations. In addition, the fold changes of the T-MF mutation frequency of the TK gene under ELE treatment were no greater than three times in comparison to solvent control, indicating that ELE did not cause TK gene mutation. Therefore, we concluded that ELE does not cause genotoxicity. Since these genotoxicity studies were in keeping with the OECD guidelines, the results of no genotoxicity could not only meet the demand of China, but also be admitted by other counties, which benefited the normal trade of ELE.
3.6 Cytotoxicity Testing of ELE
To license a functional food, new material must undergo a cytotoxicity test. In this study, three typical cells (CHL, CHO, V79) were used to evaluate cytotoxicity [33]. The cell viabilities of CHL, CHO, and V79 cells under ELE treatment are listed in Table 6. The cell viability of V79 cells under ELE treatment was in a range of 65.22%–81.32%, indicating that ELE slightly induced V79 cell death. Although slight growth inhibition of ELE-treated V79 cells was determined, the inhibitory effect of ELE on CHL and CHO cells was acceptable as the cell viability of these ELE-treated cells surpassed 85%, no matter if the concentration of ELE was low or high, suggesting that ELE did not induce significant cytotoxicity. Since checking the cytotoxicity is a prerequisite to deciding the concentrations to be tested in a genotoxicity assay and also aids in the interpretation of positive results, our results can certify the concentrations used in genotoxicity tests are acceptable based on the standards and can support the obtained negative results from each test to some extent, which co-verify that ELE has no genotoxicity.
3.7 Growth Performance of Rats under Long-Term ELE Treatment
Long-term toxicity testing is essential to evaluate the in vivo safety of plant extracts for serving as functional foods [34]. It can determine whether long-term administration induces tissue and organ damage, and also can determine the safe dose of administration. In the present study, no mortality in rats was observed during the entire long-term toxicity experiment. The results of ELE on the growth of rats are listed in Table 7. Rat body weight increases under ELE treatment were observed in comparison with the control, and the body weights of male rats significantly increased (P < 0.05), indicating that ELE was growth-promoting. Additionally, the heart and kidney weights of both male and female rats increased under ELE treatment, especially under high-level treatment (B group). Surprisingly, the brain weights of male rats significantly increased under ELE treatment when compared to control rats (P < 0.05), whereas this phenomenon was not observed in female rats. No significant variations were detected in the weights of other tissues (liver, spleen, adrenal gland, and testis) in both male and female rats among the four tested groups.
Many studies have reported that dietary medical plant extracts promoted animal growth [35,36], such as Piper sarmentosum extract for weaned piglets [37], Moringa oleifera extract for goats [38], Crataegus pinnatifida extract for juvenile golden pompano [39], and Cassia abbreviata extract for chickens [40]. Our previous work also showed that dietary supplementation with E. ulmoides leaf powder enhanced the growth performance of weanling piglets [41]. Similar to weanling piglets, the body weights of rats (both male and female) under ELE treatment increased in comparison to the control group, indicating that the growth performance of rats was strongly associated with ELE. The effects of ELE on elevated animal growth performance may be ascribed to numerous active ingredients of ELE belonging to phytoestrogens, such as aucubin and wogonin [42], which can serve as estrogen receptor modulators in animals and strengthen the growth and development of animals, such as diet supplementation with ELE at a relatively high level shown to heighten the laying performance of hens [43].
3.8 Blood Index Analysis of Rats under ELE Treatment
No significant alterations in the routine blood indexes of rats (both male and female) were observed under ELE treatment when compared to the control (Table S1). An evaluation of the blood biochemistry indexes (Table 8) showed that the AST concentration in rats significantly increased under dietary supplementation with ELE when compared to the control (P < 0.05), no matter if the rats were male or female, implying that ELE influenced liver function. The blood TG and LDL-C concentrations significantly decreased and HDL-C concentrations significantly increased under high-level ELE treatment (B group), indicating that dietary addition of ELE at a relatively high level contributed to lowering the blood lipids of rats. Interestingly, the blood TC concentrations in male rats significantly decreased under high-level ELE treatment in comparison with the control, whereas the TC concentrations in female rats significantly increased, indicating a divergent mechanism of rats to cope with ELE. Additionally, four typical antioxidant indexes (SOD, GSH, MDA, CAT) and three typical inflammatory factors (TNF-α, IL-1β, IL-6) were analyzed in both male and female rats. No significant difference in antioxidant indexes or inflammatory factors was determined among the four tested groups (Table S2), suggesting that ELE did not induce oxidative injury or an inflammatory response in rats.
Research on hyperlipidemia amelioration generally focuses on plant extracts or products that positively participate in lipid metabolism, such as Ampelopsis grossedentata extract and its characteristic component (dihydromyricetin) that was found to significantly lower plasma TG, TC, and LDL-C levels and increase plasma HDL-C levels in mice and hens [43–46]. In addition, evidence has shown that E. ulmoides can also improve blood lipid levels [4]. In this research, plasma TG and LDL-C concentrations significantly decreased and the plasma HDL-C concentration significantly increased in both male and female rats under ELE treatments. Although a dose-response was not determined, the highest dose of ELE exerted a slightly stronger effect on HDL-C and LDL-C contents alterations if compared with the other two doses, which is in accordance with the findings in hens [43], indicating that ELE is beneficial for lowering blood lipid of rats. Previous research showed that the active ingredients of E. ulmoides (flavonoids and phenolics) ameliorated hypertriglyceridemia by upregulating the expression of genes related to hepatic α-, β-, and ω-oxidation [47] and thus participated in regulating blood lipid metabolism [48]. Since the complete mechanism of the lipid-decreasing effects of E. ulmoides is a complex network, the specific mechanisms should be further studied based on the abundant composition data of ELE.
3.9 Histopathological Analysis of Rats under ELE Treatment
Five tissues (liver, spleen, kidney, stomach, and pancreas) of rats were selected for conducting histopathological examinations (Fig. 1). Among the four groups, no significant changes or cytoplasmic vacuolation were observed. The intercellular spaces of the liver, spleen, kidney, stomach, and pancreas revealed no significant differences. The cell morphology of the liver, spleen, kidney, stomach, and pancreas under ELE treatment was regular without abnormal cellular changes when compared to the control; for example, the glomerulus was intact without distension. No inflammatory infiltration was observed in the cells of tissues under ELE treatment. Therefore, it was concluded that ELE did not cause a histopathological lesion in rats.
Figure 1: Effect of dietary supplementation E. ulmoides leaf extract (ELE) on the selected tissues of rats. (A−D) Representative photomicrographs of liver (20×), spleen (10×), kidney (20×), pancreas (10×), and stomach (20×) among the 4 tested groups
In our study, the long-term toxicity tests showed that the growth performance of rats (both male and female) were unaffected. The routine blood indexes, the antioxidant indexes, and the inflammatory factors were consistent between the ELE treatment and the control, indicating that long-term ingestion of ELE was harmless to the body because oxidative injury and inflammatory responses were not induced. No visible difference in the liver, spleen, kidney, stomach, or pancreas was identified between the ELE treatment and the control, which indicated that ELE did not cause histopathological lesions in rats. Taken together, we can therefore conclude that ELE does not cause long-term in vivo toxicity.
The duodenum villus heights of rats in the A group (both male and female) were significantly lower than those of rats under ELE treatment (Fig. 2), especially in female rats (P < 0.05), indicating that ELE promoted intestinal villus growth. No significant differences in the duodenum crypt depth of rats (both male and female) were observed between the ELE treatment groups and the A group (P > 0.05). A slight downregulation in the ileum villus height and crypt depth of female rats was observed under ELE treatment in comparison with the control condition (A group), without a significant difference (P > 0.05). In addition, the ileum villus height and crypt depth of male rats showed no difference among the four tested groups. In accordance with the alterations in villus height and crypt depth, the villus height to crypt depth ratio of the duodenum and ileum also changed. The ratios of the A group were slightly lower than the ratios of the B, C, and D groups without significant differences (P > 0.05). These results implied that ELE was beneficial to intestinal morphology.
Figure 2: Effect of dietary supplementation E. ulmoides leaf extract (ELE) on the selected intestinal of rats. (A−D) Representative photomicrographs of villus in the duodenum (10×) and ileum (20×) of the 4 tested groups. (E) Villus height, crypt depth, and villus height to crypt depth ratio of duodenum and ileum in the 4 tested groups. Different small letters indicate significant differences at P < 0.05 level of tukey test under different treatments
Based on intestinal histomorphology, a higher villus height, as well as a higher villus height to crypt depth ratio, contribute to nutrient absorption [49,50]. Consistently, a higher villus height together with a higher villus height to crypt depth ratio of the duodenum and ileum were found under ELE treatment in comparison with the control condition, suggesting that dietary supplementation with ELE at a relatively high level was beneficial to nutrient absorption, as determined by intestinal histology changes. Therefore, another reason the growth performance was elevated may be attributed to ELE-mediated enhanced nutrient absorption via intestinal morphological changes. Furthermore, the alterations in villus height and crypt depth of female rats were more obvious than those of male rats, suggesting that ELE functioned as a phytoestrogen in stimulating the growth performance of rats.
3.10 Antimicrobial Testing of ELE
To further investigate the bioactivities of ELE, the antimicrobial activities, anticancer capacity, and antioxidant abilities of ELE were explored. The inhibition rates of ELE against E. coli, S. aureus, P. aeruginosa, and A. niger are listed in Table 9. Based on the results, ELE exhibited good to excellent antimicrobial activities against S. aureus and P. aeruginosa as the inhibition rate against S. aureus reached above 80% and the inhibition rate against P. aeruginosa ranged from 58.93%–98.19%. A moderate inhibition rate of ELE against E. coli and A. niger was noted as the inhibition rates were in a range of 34.08%–58.30%. These results imply that ELE has certain antimicrobial activity, which is in accordance with the former report [4].
3.11 Potential Anticancer Capacity of ELE
The cell viability of HepG2 cells under different ELE treatments was evaluated (Fig. 3). As the concentration of ELE increased, the viability of HepG2 cells decreased, which was in agreement with the effects of ELE on V79 cells. Besides, the IC50 of ELE against HepG2 was 3.98 mg/L, which was significantly lower than the IC50 of cisplatin (12.52 mg/L). Although the experimental design was the same as in cytotoxicity assays and the treatment time was only 12 h, a down-regulation in cells viability of HepG2 was observed along with ELE concentration increasing, suggesting ELE had the potential in inhibiting HepG2 cells proliferation based on the numerous active components.
Figure 3: The cell viability of HepG-2 cells under different E. ulmoides leaf extract (ELE) treatment. DDP represents cisplatin
3.12 Free Radical Scavenging Rate of ELE
Four typical free radicals (⋅OH, DPPH⋅, PTIO⋅, ABTS⋅) were chosen to assess the in vitro free radical scavenging rate of ELE (Fig. 4). Although scavenging rates (%) of ELE against the selected free radicals were lower than that of vitamin C, the free radical scavenging rate of ELE against ⋅OH, DPPH⋅, PTIO⋅, and ABTS⋅ all increased as the ELE concentration increased, indicating that ELE at a relatively high concentration was beneficial for free radical scavenging. Due to flavonoids of E. ulmoides leaves (e.g., CGA, rutin, ferulic acid, and caffeic acid) possessing good antioxidant activity [15], our results certified the ELE could also reveal preferable antioxidant activities, which had benefits in anti-aging [23].
Figure 4: The free radical scavenging rates of E. ulmoides leaf extract (ELE). VC represents vitamin C
Because several types of active compounds were identified in E. ulmoides (either the bark or leaves) [5], E. ulmoides has been found to exhibit multiple pharmacological activities [4,51]. Numerous studies have shown that CGA, the most important characteristic component of E. ulmoides leaves, protected mice against Cd-induced hepatorenal injury [52], and the other characteristic components with high levels in E. ulmoides leaves, such as geniposidic acid (GPA) and aucubin (AU), caused free radical scavenging and anticancer effects [53–55]. Therefore, these results are corroborative evidence showing that ELE has significant prospects for antimicrobial, anticancer, and antioxidant uses, based on the active ingredients. Meanwhile, our previous studies had proved E. ulmoides leaf extract revealed higher antihypertension effect than bark extract, and could enhance the body health and production of hens [18,43]. Together with our previous research showing that GPA and AU have tremendous potential for their anti-aging effects [23], ELE also shows outstanding potential in traditional Chinese health food fields, such as food, medicine, feeding, and daily chemical product. Many types of products derived from ELE have emerged, such as tea [56], beverages [57], feed additives [43], and healthy food [58]. Our work can facilitate E. ulmoides leaf inclusion in the directory of traditional Chinese health food as a new resource and can ensure the export quality of ELE.
In the present study, a comprehensive assessment of the safety of E. ulmoides leaf extract was conducted through a combination of genotoxicity tests, cytotoxicity assay, and in vivo long-term animal toxicity analysis. The conclusions from the preliminary data and discussion were as follows:
1. The reverse mutation number of nominal bacterial strains, the micronucleus rates of in vivo mammalian erythrocyte and in vitro mammalian cells, and the TFT resistance mutation frequency of the TK gene under ELE treatment were less than the rated fold change of revealing genotoxicity in comparison with the solvent control, indicating that ELE had no genotoxicity.
2. The growth performance of rats under ELE treatment was enhanced and no oxidative injury, inflammatory response, as well as histopathological lesions of tissues were detected between ELE-treated and control rats, indicating that ELE also had no in vivo long-term toxicity.
3. The blood lipid levels were ameliorated under ELE treatment, suggesting that ELE could potentially improve body health.
4. Good to excellent antimicrobial, anticancer, and antioxidant activities of ELE were determined, implying that ELE possessed preferable bioactivities.
These results could deepen our understanding of ELE as a traditional Chinese health food in addition to supporting the use of ELE in actual applications, such as the food, drug, and cosmetics industries.
Acknowledgement: This study was supported by the National Natural Science Foundation of China (Grant No. 42107020) and the Scientific Research Start Funds, Hunan Institute of Technology (No. HQ20014). We thank LetPub (www.letpub.com) for its linguistic assistance during the preparation of this manuscript.
Funding Statement: The authors received no specific funding for this study.
Conflicts of Interest: The authors declare that they have no conflicts of interest to report regarding the present study.
References
1. Hu, S. Y. (1979). A contribution to our knowledge of Tu-chung-Eucommia ulmoides. American Journal of Chinese Medicine, 7, 5–37. https://doi.org/10.1142/S0192415X79000039 [Google Scholar] [PubMed] [CrossRef]
2. Yen, G., Hsieh, C. (2000). Reactive oxygen species scavenging activity of du-zhong (Eucommia ulmoides Oliv.) and its active compounds. Journal of Agricultural and Food Chemistry, 48, 3431–3436. https://doi.org/10.1021/jf000150t [Google Scholar] [PubMed] [CrossRef]
3. Cronquist, A. (1981). An integrated system of classification of flowering plants. New York: Columbia University Press. [Google Scholar]
4. Wang, C. Y., Tang, L., He, J. W., Li, J., Wang, Y. Z. (2019). Ethnobotany, phytochemistry and pharmacological properties of Eucommia ulmoides: A review. American Journal of Chinese Medicine, 47(2), 259–300. https://doi.org/10.1142/S0192415X19500137 [Google Scholar] [PubMed] [CrossRef]
5. Zhu, M. Q., Sun, R. C. (2018). Eucommia ulmoides oliver: A potential feedstock for bioactive products. Journal of Agricultural and Food Chemistry, 66, 5433–5438. https://doi.org/10.1021/acs.jafc.8b01312 [Google Scholar] [PubMed] [CrossRef]
6. Chinese Pharmacopoeia Commission (2010). Chinese pharmacopoeia, pp. 154–155. Beijing, China: China Medical Science and Technology Press. [Google Scholar]
7. He, X. R., Wang, J. H., Li, M. X., Hao, D. J., Yang, Y. et al. (2014). Eucommia ulmoides Oliv.: Ethnopharmacology, phytochemistry and pharmacology of an important traditional Chinese medicine. Journal of Ethnopharmacology, 151, 78–92. https://doi.org/10.1016/j.jep.2013.11.023 [Google Scholar] [PubMed] [CrossRef]
8. Li, Q., Feng, Y., He, W., Wang, L., Wang, R. et al. (2017). Post-screening characterisation and in vivo evaluation of an antiinflammatory polysaccharide fraction from Eucommia ulmoides. Carbohydrate Polymers, 169, 304–314. https://doi.org/10.1016/j.carbpol.2017.04.034 [Google Scholar] [PubMed] [CrossRef]
9. Hosoo, S., Koyama, M., Watanabe, A., Ishida, R., Hirata, T. et al. (2017). Preventive effect of eucommia leaf extract on aortic media hypertrophy in wistar-kyoto rats fed a high-fat diet. Hypertension Research, 40, 546–551. https://doi.org/10.1038/hr.2016.189 [Google Scholar] [PubMed] [CrossRef]
10. Cho, S., Hong, R., Yim, P., Yeom, M., Lee, B. et al. (2018). An herbal formula consisting of Schisandra chinensis (Turcz.) Baill, Lycium chinense Mill and Eucommia ulmoides Oliv alleviates disuse muscle atrophy in rats. Journal of Ethnopharmacology, 213, 328–339. https://doi.org/10.1016/j.jep.2017.10.008 [Google Scholar] [PubMed] [CrossRef]
11. Huang, Q., Zhang, F., Liu, S., Jiang, Y., Ouyang, D. (2021). Systematic investigation of the pharmacological mechanism for renal protection by the leaves of Eucommia ulmoides Oliver using UPLC-Q-TOF/MS combined with network pharmacology analysis. Biomedicine & Pharmacotherapy, 140, 111735. https://doi.org/10.1016/j.biopha.2021.111735 [Google Scholar] [PubMed] [CrossRef]
12. Xing, Y. F., He, D., Wang, Y., Zeng, W., Zhang, C. et al. (2019). Chemical constituents, biological functions and pharmacological effects for comprehensive utilization of Eucommia ulmoides Oliver. Food Science and Human Wellness, 8, 177–188. https://doi.org/10.1016/j.fshw.2019.03.013 [Google Scholar] [CrossRef]
13. Kwon, S. H., Lee, H. K., Kim, J. A., Hong, S. I., Kim, S. Y. et al. (2011). Neuroprotective effects of Eucommia ulmoides Oliv. Bark on amyloid beta (25–35)-induced learning and memory impairments in mice. Neuroscience Letters, 487, 123–127. https://doi.org/10.1016/j.neulet.2010.10.042 [Google Scholar] [PubMed] [CrossRef]
14. Nakazawa, Y., Takeda, T., Suzuki, N., Hayashi, T., Harada, Y. et al. (2013). Histochemical study of trans-polyisoprene accumulation by spectral confocal laser scanning microscopy and a specific dye showing fluorescence solvatochromism in the rubber-producing plant, Eucommia ulmoides Oliver. Planta, 238, 549–560. https://doi.org/10.1007/s00425-013-1912-2 [Google Scholar] [PubMed] [CrossRef]
15. Kulomaa, A., Sir´en, H., Riekkola, M. L. (1997). Identification of antioxidative compounds in plant beverages by capillary electrophoresis with the marker index technique. Journal of Chromatography A, 781, 523–532. https://doi.org/10.1016/S0021-9673(97)00357-9 [Google Scholar] [CrossRef]
16. Hussain, T., Tan, B., Liu, G., Oladele, O. A., Rahu, N. et al. (2016). Health-promoting properties of Eucommia ulmoides: A review. Evidence-Based Complementary and Alternative Medicine, 2016, 5202908. [Google Scholar] [PubMed]
17. Huang, L., Lyu, Q., Zheng, W., Yang, Q., Cao, G. (2021). Traditional application and modern pharmacological research of Eucommia ulmoides Oliv. Chinese Medicine, 16(1), 73. https://doi.org/10.1186/s13020-021-00482-7 [Google Scholar] [PubMed] [CrossRef]
18. Wang, X. S., Peng, M. J., He, C. T. (2022). The antihypertensive effects of Eucommia ulmoides leaf water/ethanol extracts are chlorogenic acid dependent. Journal of Functional Foods, 94, 105129. https://doi.org/10.1016/j.jff.2022.105129 [Google Scholar] [CrossRef]
19. Zhao, Y., Wang, J., Ballevre, O., Luo, H., Zhang, W. (2012). Antihypertensive effects and mechanisms of chlorogenic acids. Hypertension Research, 35(4), 370–374. https://doi.org/10.1038/hr.2011.195 [Google Scholar] [PubMed] [CrossRef]
20. Onakpoya, I. J., Spencer, E. A., Thompson, M. J., Heneghan, C. J. (2015). The effect of chlorogenic acid on blood pressure: A systematic review and meta-analysis of randomized clinical trials. Journal of Human Hypertension, 29(2), 77–81. https://doi.org/10.1038/jhh.2014.46 [Google Scholar] [PubMed] [CrossRef]
21. Hakkou, Z., Maciuk, A., Leblais, V., Bouanani, N. E., Mekhfi, H. et al. (2017). Antihypertensive and vasodilator effects of methanolic extract of Inula viscosa: Biological evaluation and POM analysis of cynarin, chlorogenic acid as potential hypertensive. Biomedicine & Pharmacotherapy, 93, 62–69. https://doi.org/10.1016/j.biopha.2017.06.015 [Google Scholar] [PubMed] [CrossRef]
22. Suzuki, A., Kagawa, D., Ochiai, R., Tokimitsu, I., Saito, I. (2002). Green coffee bean extract and its metabolites have a hypotensive effect in spontaneously hypertensive rats. Hypertension Research, 25(1), 99–107. https://doi.org/10.1291/hypres.25.99 [Google Scholar] [PubMed] [CrossRef]
23. Wang, X., Wang, Z., Yang, Q., Peng, S., Peng, M. (2022). Determining the in vitro anti-aging effect of the characteristic components from Eucommia ulmoides. Journal of Renewable Materials, 10(12), 3131–3145. https://doi.org/10.32604/jrm.2022.020204 [Google Scholar] [CrossRef]
24. Li, X., Xie, H., Jiang, Q., Wei, G., Lin, L. et al. (2017). The mechanism of (+) taxifolin’s protective antioxidant effect for •OH-treated bone marrow-derived mesenchymal stem cells. Cellular & Molecular Biology Letters, 22, 31. https://doi.org/10.1186/s11658-017-0066-9 [Google Scholar] [PubMed] [CrossRef]
25. Dai, X., Huang, Q., Zhou, B., Gong, Z., Liu, Z. et al. (2013). Preparative isolation and purification of seven main antioxidants from Eucommia ulmoides Oliv. (Du-zhong) leaves using HSCCC guided by DPPH-HPLC experiment. Food Chemistry, 139, 563–570. [Google Scholar] [PubMed]
26. Feng, H., Fan, J., Song, Z., Du, X., Chen, Y. et al. (2016). Characterization and immunoenhancement activities of Eucommia ulmoides polysaccharides. Carbohydrate Polymers, 136, 803–811. https://doi.org/10.1016/j.carbpol.2015.09.079 [Google Scholar] [PubMed] [CrossRef]
27. Zhou, Y., Liang, M., Li, W., Li, K., Li, P. et al. (2009). Protective effects of Eucommia ulmoides Oliv. Bark and leaf on amyloid β-induced cytotoxicity. Environmental Toxicology and Pharmacology, 28, 342–349. https://doi.org/10.1016/j.etap.2009.05.012 [Google Scholar] [PubMed] [CrossRef]
28. Wang, L., Zhao, F., Liu, K. (2008). Advances in studies on pharmacological effect of arctiin and arctigenin. Chinese Traditional and Herbal Drugs, 39, 467–470. [Google Scholar]
29. Nicolette, J., Dakoulas, E., Pant, K., Crosby, M., Kondratiuk, A. et al. (2018). A comparison of 24 chemicals in the six-well bacterial reverse mutation assay to the standard 100-mm petri plate bacterial reverse mutation assay in two laboratories. Regulatory Toxicology and Pharmacology, 100, 134–160. https://doi.org/10.1016/j.yrtph.2018.10.005 [Google Scholar] [PubMed] [CrossRef]
30. Igl, B. W., Bitsch, A., Bringezu, F., Chang, S., Dammann, M. et al. (2019). The rat bone marrow micronucleus test: Statistical considerations on historical negative control data. Regulatory Toxicology and Pharmacology, 102, 13–22. https://doi.org/10.1016/j.yrtph.2018.12.009 [Google Scholar] [PubMed] [CrossRef]
31. Baderna, D., Gadaleta, D., Lostaglio, E., Selvestrel, G., Raitano, G. et al. (2020). New in silico models to predict in vitro micronucleus induction as marker of genotoxicity. Journal of Hazardous Materials, 385, 121638. https://doi.org/10.1016/j.jhazmat.2019.121638 [Google Scholar] [PubMed] [CrossRef]
32. Sassa, A., Fukuda, T., Ukai, A., Nakamura, M., Sato, R. et al. (2021). Follow-up genotoxicity assessment of Ames-positive/equivocal chemicals using the improved thymidine kinase gene mutation assay in DNA repair-deficient human TK6 cells. Mutagenesis, 36(5), 331–338. https://doi.org/10.1093/mutage/geab025 [Google Scholar] [PubMed] [CrossRef]
33. Miller, B., Pötter-Locher, F., Seelbach, A., Stopper, H., Utesch, D. et al. (1998). Evaluation of the in vitro micronucleus test as an alternative to the in vitro chromosomal aberration assay: Position of the GUM working group on the in vitro micronucleus test. Gesellschaft für Umwelt-Mutations-forschung. Mutation Research/Reviews in Mutation Research, 410(1), 81–116. https://doi.org/10.1016/S1383-5742(97)00030-6 [Google Scholar] [PubMed] [CrossRef]
34. Antonelli-Ushirobira, T. M., Blainski, A., Fernandes, H. G., Moura-Costa, G. F., Costa, M. A. et al. (2015). Acute toxicity and long-term safety evaluation of the crude extract from rhizomes of Limonium brasiliense in mice and rats. Journal of Ethnopharmacology, 174, 293–298. https://doi.org/10.1016/j.jep.2015.08.022 [Google Scholar] [PubMed] [CrossRef]
35. Farahat, M., Ibrahim, D., Kishawy, A. T. Y., Abdallah, H. M., Hernandez-Santana, A. et al. (2021). Effect of cereal type and plant extract addition on the growth performance, intestinal morphology, caecal microflora, and gut barriers gene expression of broiler chickens. Animal, 15(3), 100056. https://doi.org/10.1016/j.animal.2020.100056 [Google Scholar] [PubMed] [CrossRef]
36. Sun, Z., Liu, Y., Wei, Z., Mai, H., Liu, Q. et al. (2021). The effects of dietary compound plant extracts on growth performance, liver and intestine health, and immune related genes expression in hybrid grouper (Epinephelus lanceolatus♂ × Epinephelus fuscoguttatus♀). Fish and Shellfish Immunology, 119, 11–18. https://doi.org/10.1016/j.fsi.2021.09.013 [Google Scholar] [PubMed] [CrossRef]
37. Wang, D. F., Zhou, L. L., Zhou, H. L., Hou, G. Y., Zhou, X. et al. (2017). Effects of Piper sarmentosum extract on the growth performance, antioxidant capability and immune response in weaned piglets. Journal of Animal Physiology and Animal Nutrition, 101(1), 105–112. https://doi.org/10.1111/jpn.12517 [Google Scholar] [PubMed] [CrossRef]
38. Pedraza-Hernández, J., Elghandour, M. M. M. Y., Khusro, A., Salem, M. Z. M., Camacho-Diaz, L. M. et al. (2021). Assessment on bioactive role of Moringa oleifera leaves as anthelmintic agent and improved growth performance in goats. Tropical Animal Health and Production, 53(2), 318. https://doi.org/10.1007/s11250-021-02745-9 [Google Scholar] [PubMed] [CrossRef]
39. Tan, X., Sun, Z., Huang, Z., Zhou, C., Lin, H. et al. (2017). Effects of dietary hawthorn extract on growth performance, immune responses, growth- and immune-related genes expression of juvenile golden pompano (Trachinotus ovatus) and its susceptibility to Vibrio harveyi infection. Fish and Shellfish Immunology, 70, 656–664. https://doi.org/10.1016/j.fsi.2017.09.041 [Google Scholar] [PubMed] [CrossRef]
40. Jobe, M. C., Ncobela, C. N., Kunene, N. W., Opoku, A. R. (2019). Effects of Cassia abbreviata extract and stocking density on growth performance, oxidative stress and liver function of indigenous chickens. Tropical Animal Health and Production, 51(8), 2567–2574. https://doi.org/10.1007/s11250-019-01979-y [Google Scholar] [PubMed] [CrossRef]
41. Peng, M., Wang, Z., Peng, S., Zhang, M., Duan, Y. et al. (2019). Dietary supplementation with the extract from Eucommia ulmoides leaves changed epithelial restitution and gut microbial community and composition of weanling piglets. PLoS One, 14(9), e0223002. https://doi.org/10.1371/journal.pone.0223002 [Google Scholar] [PubMed] [CrossRef]
42. Wang, H., Li, M. C., Yan, J., Yang, D., Su, Y. F. et al. (2011). Estrogenic properties of six compounds derived from Eucommia ulmoides Oliv. and their differing biological activity through estrogen receptors α and β. Food Chemistry, 129(2), 408–416. https://doi.org/10.1016/j.foodchem.2011.04.092 [Google Scholar] [PubMed] [CrossRef]
43. Peng, M. J., Huang, T., Yang, Q. L., Peng, S., Jin, Y. X. et al. (2022). Dietary supplementation Eucommia ulmoides extract at high content served as a feed additive in the hens industry. Poultry Science, 101(3), 101650. https://doi.org/10.1016/j.psj.2021.101650 [Google Scholar] [PubMed] [CrossRef]
44. Liu, T. T., Zeng, Y., Tang, K., Chen, X., Zhang, W. et al. (2017). Dihydromyricetin ameliorates atherosclerosis in LDL receptor deficient mice. Atherosclerosis, 262, 39–50. https://doi.org/10.1016/j.atherosclerosis.2017.05.003 [Google Scholar] [PubMed] [CrossRef]
45. Fan, L., Qu, X., Yi, T., Peng, Y., Jiang, M. et al. (2020). Metabolomics of the protective effect of Ampelopsis grossedentata and its major active compound dihydromyricetin on the liver of high-fat diet hamster. Evidence-based Complementary and Alternative Medicine, 35, 1–15. https://doi.org/10.1155/2020/3472578 [Google Scholar] [PubMed] [CrossRef]
46. Huang, T., Wang, X., Yang, Q., Peng, S., Peng, M. (2022). Effects of dietary supplementation with Ampelopsis grossedentata extract on production performance and body health of hens. Tropical Animal Health and Production, 54(1), 45. https://doi.org/10.1007/s11250-022-03044-7 [Google Scholar] [PubMed] [CrossRef]
47. Kobayashi, Y., Hiroi, T., Araki, M., Hirokawa, T., Miyazawa, M. et al. (2012). Facilitative effects of Eucommia ulmoides on fatty acid oxidation in hypertriglyceridaemic rats. Journal of the Science of Food and Agriculture, 92(2), 358–365. https://doi.org/10.1002/jsfa.4586 [Google Scholar] [PubMed] [CrossRef]
48. Gong, M., Su, C., Fan, M., Wang, P., Cui, B. et al. (2022). Mechanism by which Eucommia ulmoides leaves regulate nonalcoholic fatty liver disease based on system pharmacology. Journal of Ethnopharmacology, 282, 114603. https://doi.org/10.1016/j.jep.2021.114603 [Google Scholar] [PubMed] [CrossRef]
49. Ege, G., Bozkurt, M., Koçer, B., Tüzün, A. E., Uygun, M. et al. (2019). Influence of feed particle size and feed form on productive performance, egg quality, gastrointestinal tract traits, digestive enzymes, intestinal morphology, and nutrient digestibility of laying hens reared in enriched cages. Poultry Science, 98, 3787–3801. https://doi.org/10.3382/ps/pez082 [Google Scholar] [PubMed] [CrossRef]
50. Chen, F., Zhang, H., Du, E., Jin, F., Zheng, C. et al. (2021). Effects of magnolol on egg production, egg quality, antioxidant capacity, and intestinal health of laying hens in the late phase of the laying cycle. Poultry Science, 100, 835–843. https://doi.org/10.1016/j.psj.2020.10.047 [Google Scholar] [PubMed] [CrossRef]
51. Gu, T., Li, G., Wu, X., Zeng, T., Xu, Q. et al. (2020). Effects of immunopotentiators on biochemical parameters, proinflammatory cytokine, and nonspecific immune responses in Shaoxing ducklings. Poultry Science, 99, 5461–5471. https://doi.org/10.1016/j.psj.2020.08.069 [Google Scholar] [PubMed] [CrossRef]
52. Ding, Y., Li, X., Liu, Y., Wang, S., Cheng, D. (2021). Protection mechanisms underlying oral administration of chlorogenic acid against cadmium-induced hepatorenal injury related to regulating intestinal flora balance. Journal of Agricultural and Food Chemistry, 69, 1675–1683. https://doi.org/10.1021/acs.jafc.0c06698 [Google Scholar] [PubMed] [CrossRef]
53. Jaisankar, A. I., Arivarasu, L., Rajeshkumar, S. (2017). Free radical scavenging and anti-inflammatory activity of chlorogenic acid mediated silver nanoparticle. Natural Products and Bioprospecting, 7, 335–404. [Google Scholar]
54. Háznagy-Radnai, E., Wéber, E., Czigle, S., Berkecz, R., Csedő, K. et al. (2014). Identification of iridoids, flavonoids and triterpenes from the methanolic extract of melampyrum bihariense A. Kern. and the antioxidant activity of the extract. Chromatographia, 77, 1153–1159. https://doi.org/10.1007/s10337-014-2672-2 [Google Scholar] [CrossRef]
55. Kim, S. H., Lee, J. C. (2021). Quercetin protects hepatocytes against CCl4-induced apoptosis via SIRT1 regulation. Cell and Tissue Biology, 15(4), 381–387. https://doi.org/10.1134/S1990519X21040039 [Google Scholar] [CrossRef]
56. Nakamura, T., Nakazawa, Y., Onizuka, S., Satoh, S., Chiba, A. et al. (1997). Antimutagenicity of tochu tea (an aqueous extract of Eucommia ulmoides leaves1. The clastogen-suppressing effects of tochu tea in CHO cells and mice. Mutation Research-Genetic Toxicology and Environmental Mutagenesis, 388(1), 7–20. https://doi.org/10.1016/S1383-5718(96)00096-4 [Google Scholar] [PubMed] [CrossRef]
57. Takamura, C., Hirata, T., Ueda, T., Ono, M., Miyashita, H. et al. (2007). Iridoids from the green leaves of Eucommia ulmoides. Journal of Natural Products, 70(8), 1312–1316. https://doi.org/10.1021/np0780046 [Google Scholar] [PubMed] [CrossRef]
58. Zhang, Z. S., Liu, Y. L., Che, L. M. (2018). Characterization of a new α-Linolenic acid-rich oil: Eucommia ulmoides seed oil. Journal of Food Science, 83(3), 617–623. https://doi.org/10.1111/1750-3841.14049 [Google Scholar] [PubMed] [CrossRef]
Figure S1:: Chromatograms of standards. (A) and characteristic components in E. ulmoides leaf extract (ELE) (B) using HPLC system.
Cite This Article
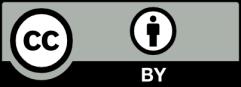