Open Access
ARTICLE
Application of Zinc, Iron and Boron Enhances Productivity and Grain Biofortification of Mungbean
1 Agronomic Research Institute, Ayub Agricultural Research Institute Faisalabad, Faisalabad, 38000, Pakistan
2 Soil and Water Testing Laboratory, Ayub Agricultural Research Institute Faisalabad, Faisalabad, 38000, Pakistan
3 Department of Plant Sciences, Quaid-i-Azam University, Islamabad, 45320, Pakistan
4 Department of Agricultural Engineering, Khwaja Fareed University of Engineering and Technology, Rahim Yar Khan, 64200, Pakistan
5 College of Agronomy, Northwest A&F University, Yangling, 712100, China
6 Plant Pathology Research Institute, Faisalabad, 38000, Pakistan
7 Department of Zoology, College of Science, King Saud University, Riyadh, 11451, Saudi Arabia
8 Department of Biotechnology, Yeungnam University, Gyeongsan, 38641, Korea
* Corresponding Authors: Basharat Ali. Email: ; Sadam Hussain. Email:
(This article belongs to the Special Issue: Physiological and Molecular Interventions in Improving Abiotic Stress Tolerance in Plants)
Phyton-International Journal of Experimental Botany 2023, 92(4), 983-999. https://doi.org/10.32604/phyton.2023.025813
Received 01 August 2022; Accepted 12 September 2022; Issue published 06 January 2023
Abstract
Deficiencies of essential vitamins, iron (Fe), and zinc (Zn) affect over one-half of the world’s population. A significant progress has been made to control micronutrient deficiencies through supplementation, but new approaches are needed, especially to reach the rural poor. Agronomic biofortification of pulses with Zn, Fe, and boron (B) offers a pragmatic solution to combat hidden hunger instead of food fortification and supplementation. Moreover, it also has positive effects on crop production as well. Therefore, we conducted three separate field experiments for two consecutive years to evaluate the impact of soil and foliar application of the aforementioned nutrients on the yield and seed biofortification of mungbean. Soil application of Zn at 0, 4.125, 8.25, Fe at 0, 2.5, 5.0 and B at 0, 0.55, 1.1 kg ha−1 was done in the first, second and third experiment, respectively. Foliar application in these experiments was done at 0.3% Zn, 0.2% Fe and 0.1% B respectively one week after flowering initiation. Data revealed that soil-applied Zn, Fe and B at 8.25, 5.0 and 1.1 kg ha−1, respectively, enhanced the grain yield of mungbean; however, this increase in yield was statistically similar to that recorded with Zn, Fe and B at 4.125, 2.5 and 0.55 kg ha−1, respectively. Foliar application of these nutrients at flower initiation significantly enhanced the Zn contents by 28% and 31%, Fe contents by 80% and 78%, while B contents by 98% and 116% over control during 2019 and 2020, respectively. It was concluded from the results that soil application of Zn, Fe, and B enhanced the yield performance of mungbean; while significant improvements in seed Zn, Fe, and B contents were recorded with foliar application of these nutrients.Keywords
Mungbean, being recognized as poor man’s meat, contains a sufficient amount of quality proteins [1]. Its nutritional profile revealed that its seed contains 367 mg phosphorus and 132 mg calcium per 100 g of seed; whereas, carbohydrates, protein, ash, fibre and fats are 50%, 26%, 4%–5%, 3%–4.5% and 3%, respectively [2]. It is usually grown in semi-arid and arid regions of the world [3]. It is considered the main pulse crop of Pakistan and was cultivated on an area of 0.231 million hectares with a total production of 0.204 million tonnes [4]. Its average yield (0.88 tonnes ha−1) is however far less than the developing countries. Improving the yield of this vital crop both quantitatively as well as qualitatively can further make it more profitable economically and beneficial from a health’s point of view.
Micronutrients, though required in smaller amounts, are necessary for human health, normal plant growth and the development of all crops [5,6]. A little quantity of micronutrients is needed for better growth and production of all crop plants [7]. Shenkin [8] suggested the application of Zn, B, Se, and Fe to be beneficial in improving the immune system of plants besides increasing their growth and development. Micronutrient malnutrition is threatening the world’s population, especially in developing countries [9]. Approximately 2 billion people across the globe are Zn deficient [10] and its deficiency ranks 5th in causing deaths in developing parts of the world while 11th in the overall world [11]. About 33% of children and 40% of mothers in Pakistan are suffering from Zn deficiency, particularly in the rural areas [12]. Fe deficiency is also a global issue that is affecting about 2 billion of the world’s population especially children and women in Latin America, South Asia, and Africa [13]. About 40% of women in their reproductive age are anemic in South Asia which constitutes 37.5% of global anemic cases [14]. While in South America, the Caribbean and Central America about 46.2%, 42.9%, and 33.9% population respectively is anemic with more prevalence in children under 11 months of age [15]. Bioavailable Zn is deficient in about 50% of the world’s cultivated land [16]. In Pakistan, the situation is even worse with the prevalence of Zn deficiency in 70% of the soils [17]. The prolonged scarcity of Zn leads to poor vegetation, sexual development and low Zn contents in grain [18], reduction in leaf size and internodal length [19]. Zn deficiency in humans is directly linked with the soil’s Zn deficiency [20]. Fe is not deficient in our soils but is less bio-available due to very little accessibility to plants in readily available form (ferric form, Fe3+) and high pH of the soil [21]. In calcareous soils, low Fe bioavailability and chemical solubility are serious problems for crop plants [22]. Pakistani soils (approximately 82%) are also deficient in B. Its availability is affected by high soil pH, calcareousness and low organic matter [23]. Zn is necessary for plants as it plays an important role in enzyme activation and nucleic acid, protein, carbohydrates and lipids metabolism [24]. It improves plant photosynthesis, growth, nitrogen fixation and ultimately the yield of crops [25]. After nitrogen and phosphorus, Zn deficiency ranks third in Pakistan in limiting plant performance [26]. Zn foliar application at flowering and grain filling enhanced pod’s number, pod’s weight, seeds per pod, biological yield, 100-seed weight, and seed yield as compared to control [27]. Negative effects of Zn deficiency in humans are pronounced on immunity, bones, skin, brain and reproductive system [28] causing several disorders including cancer, diarrhea, and pneumonia [29]. Humans consuming Zn deficient food products may suffer from Zn deficiency-related health issues such as poor birth outcome, susceptibility to diseases, stunted growth, enhanced mortality, poor immune system and brain functioning [30,31]. Rehman et al. [32] suggested the economical, feasible and sustainable approach for overcoming Zn deficiency in human food which is the biofortification of Zn in grains to increase the concentration and bioavailability of Zn in food.
Iron is an essential part of hemoglobin and myoglobin proteins which provide oxygen from lungs to tissues and muscles respectively [33,34]. Fe is an essential component for the growth, development, and synthesis of connective tissues and hormones for normal cellular functioning [34]. About half the population of women and preschool children in Asia and Africa is suffering from Fe deficiency anemia (IDA) [35]. IDA reduces immunity and physical as well as cognitive development. It enhances the risk of perinatal and maternal mortality and also affects the work performance of all age groups individuals [36]. In plants, B is essential for carbohydrate and RNA metabolism, sugar transport, membrane transport, bioaccumulation of essential elements and respiration [37]. The cell wall of all the plants is strengthened by minute amounts of B compounds [38]. Some biological functions, i.e., calcium and insulin metabolism, bone growth and maintenance and life cycle completion in humans are supported by Hunt [39]. Boron actively accumulates in bones instead of soft tissues, so it is involved in the calcification and maintenance of bones and has a positive influence on the central nervous system, maintaining structural integrity and cell membrane functions [37]. B compounds have anti-inflammatory, and antioxidant properties [40]. The deficiency of B causes several pathological disorders such as Cancer and several forms of osteoarthritis and osteoporosis [41].
In a bid to cater the food needs of an ever-increasing human population, food quality and human health have been overlooked [42]. To overcome micronutrient deficiency for quality food production, two approaches are suggested in agriculture. A sustainable and long-term solution to the problem is the development of cultivars with high micronutrient concentration in the sink by breeding and genetic engineering; however, this approach takes too much time and expertise for the solution. The other well-known strategy is agronomic bio-fortification by soil and foliar application of these micronutrients [43]. To combat Zn deficiency in cereals, several foliar sprays of dilute Zn solutions are recommended from booting to grain initiation stage, due to partial phloem mobility and possible leaf burning by the toxicity of excessive Zn [44]. Hussan et al. [45] suggested that soil application of Zn can enhance the yield but the foliar application is a better option to enhance Zn concentration in grains.
A significant progress has been made to control micronutrient deficiencies through supplementation, but some of these approaches are too costly and time consuming. A substantial approach has been adopted to invest $192 million for the biofortification of Fe in beans, especially in the food system of Latin America, East Africa, and South Asia [46]. Harvest-Plus and Pan Africa Bean Research Alliance (PABRA) has claimed a “Nutrition success story” in biofortification of Bean with Fe, by citing a trial with human efficacy [47]. Due to the limited resources of rural families which cannot change their traditional eating habits biofortification is the only approach to be believed to have the ability to target these families [48]. Keeping in view the above facts and the need for biofortification in mungbean, the current study was thus planned to enhance the concentration of Zn, Fe and B in grain and mungbean productivity by hypothesizing that these micronutrients will play a key role in several metabolic processes, enzyme activation, crop growth and development and will also enhance the concentration of these nutrients in source which will ultimately be remobilized to sink.
To evaluate the impacts of Zn, Fe and B on mungbean, three different experiments were carried out with soil and foliar application (% solution w/v) of each nutrient individually at the research farm of Cereals and Pulses Section of Agronomic Research Institute, Faisalabad using randomized complete block design (RCBD) and replicating each experiment thrice. The first experiment comprised of Zn with five treatments viz. control (no Zn application), soil application of Zn at 4.125 kg ha−1, soil application of Zn at 8.25 kg ha−1, foliar application of Zn at 0.3% at flowering initiation, and foliar application of Zn at 0.3% at one week after flowering initiation. The second experiment comprised of Fe with five treatments viz. control (no Fe application), soil application of Fe at 2.5 kg ha−1, soil application of Fe at 5.0 kg ha−1, foliar application of Fe at 0.2% at flowering initiation, and foliar application of Fe at 0.2% at one week after flowering initiation. The third experiment comprised of B with five treatments viz. control (no B application), soil application of B at 0.55 kg ha−1, soil application of B at 1.1 kg ha−1, foliar application of B at 0.1% at flowering initiation and foliar application of B at 0.1% at one week after flowering initiation.
The field was cultivated twice at field capacity, rotavated, planked, and again cultivated once for seedbed preparation. Mungbean was sown in 60 cm apart rows with help of a hand drill on 30.05.2019 and 04.05.2020 during the first and second year of study, respectively. NPK at 23:58:30 kg ha−1 was applied to the crop at sowing as a basal dose. Soil application of micronutrients was done with first irrigation and foliar application was done at flower initiation using 300 liters of solution per hectare.
Soil analysis of the experimental site was done before sowing and the results are presented in Table 1.
2.2 Weather Conditions during the Research
Weather data for both years was collected from the meteorological observatory of Agronomic Research Institute Faisalabad and are presented in Fig. 1.
Figure 1: Weather data during 2019 and 2020
2.3 Data and their Collection/Analysis Procedures
Five plants from each treatment were selected and tagged at physiological maturity. After taking plant height with the help of a meter rod from the base of the soil to the tip of plants, they were cut from the base. Primary branches and the number of pods were counted per plant and averaged. Five pods were removed from each plant, their length was taken with help of a measuring scale and the numbers of seeds were counted in each pod and averaged. The crop was harvested at harvest maturity, dried, and threshed to get the seed yield. Three samples of 1,000 seeds were collected, weighed with help of a weighing balance and averaged thereof to calculate the 1,000-seed weight. Seed samples were collected and dried for grain analysis. After that, seeds were ground, sieved and used for dry ashing and wet digestion. Seed B contents were measured by dry ashing [49] and subsequent determination was done by colorimetry using Azomethine-H [50]. Seed Zn and Fe contents were determined on Atomic Absorption Spectrophotometer after wet digestion [51] of seed samples. Methods of B, Fe, and Zn determination are mentioned in ICARDA Manual by Estefan et al. [52]. Firstly, grain samples were dried at 60°C in an oven. Later, after being grounded, grains were passed through a 1 mm sieve before wet digestion. Next, the contents of B, Fe and Zn were determined based on nitric–perchloric acid digestion method.
All the recorded data were analyzed statistically using Fisher’s analysis of variance technique and the difference between treatments’ means was compared using Tukey’s Honestly Significant Difference (HSD) test [53]. Correlation (Pearson) analysis was also performed for all parameters.
3.1 Effects of Zn Application on Performance of Mungbean
Data in Table 2 shows that all the yield and yield components were enhanced by the application of Zn either through soil or foliar application during both years of study. The maximum number of primary branches, plant height, pods per plant, pod length, seeds per pod, test weight, and grain yield were recorded when Zn was applied into the soil at 8.25 kg ha−1 followed by soil application of Zn at 4.125 kg ha−1 and foliar-applied Zn at 0.3% while the minimum was recorded with control (no Zn application). In 2019, the number of primary branches was statistically more for soil-applied Zn at 8.25 kg ha−1 as compared with foliar application of 0.3% Zn at one week after flowering initiation. During the second year of the experiment, there was a non-significant difference between soil-applied Zn at 4.125 and 8.25 kg ha−1 and 0.3% foliar Zn spray for the number of primary branches at flowering initiation and one week after the flowering initiation stage. Plant height was also recorded as statistically at par when soil application of Zn was done at 8.25 and 4.125 kg ha−1 and foliar application of 0.3% Zn at one week after flowering initiation during both study years. The minimum values for the same parameter were recorded for the control without Zn application and foliar application of 0.3% Zn at flowering initiation. Similarly, Zn application at 4.125 and 8.25 kg ha−1 recorded a higher number of pods per plant with a non-significant effect between these rates. Pod length was also similar (p ≤ 0.05) for soil application of Zn at 4.125 and 8.25 kg ha−1 during both years and foliar application of 0.3% Zn in 2019. Furthermore, minimum values for pod length were recorded for the control treatment which was statistically the same as the foliar application of 0.3% Zn at both stages. The numbers of seeds per pod were statistically same for both soil and foliar application of Zn during both years of study. Soil application of Zn at 4.125 and 8.25 kg ha−1 produced maximum but statistically same values for test weight and seed yield followed by foliar application of 0.3% Zn and control without Zn application during both study years. Foliar application of 0.3% Zn produced maximum but statistically similar grain Zn concentration to soil application of Zn at 8.25 kg ha−1 during both study years followed by soil application of Zn at 4.125 kg ha−1 (Fig. 2). The minimum values for Zn concentration were recorded for the control without Zn application. A strong correlation of all parameters was found with grain yield however there was a non-significant correlation for grain Zn contents with all other parameters (Table 2A).
Figure 2: Effect of zinc (Zn) application rates on Zn contents in the grains of mungbean. T1, control; T2, soil application of Zn at 4.125 kg ha−1; T3, soil application of Zn at 8.25 kg ha−1; T4, foliar application of Zn at 0.3% at flowering initiation (FI) stage; T5, foliar application of Zn at 0.3% at one week after FI. The values are means ± SE, n = 3. Significant differences are shown by lowercase letters (at p ≤ 0.05)
3.2 Effects of Fe Application on Performance of Mungbean
Fe application increased yield and yield components of mungbean during both study years (Table 3). Our results showed that Fe application at 5.0 kg ha−1 recorded the maximum number of primary branches and pods per plant which was statistically similar to 2.5 kg ha−1 while the minimum was recorded for the control without Fe application and foliar application at 0.2% Fe at both growth stage during 2020 and both years, respectively. However, in 2019, there was a non-significant difference in the treatments. Soil application of Fe at 2.5 and 5.0 kg ha−1 and foliar application of 0.2% Fe produced statistically similar plant height while minimum values were recorded for the control treatment and foliar application of Fe at 0.2% at one week after flowering initiation during the first study year. However, in 2020, all treatments recorded statistically similar values for plant height. Similarly, for pod length seeds per pod, there was a non-significant difference among the treatments in 2019, however, Fe application at 5.0 kg ha−1 increased the same parameter in 2020 followed by soil-applied Fe at 2.5 kg ha−1. While minimum pod length and seeds per pod were recorded for control treatment which was statistically at par with foliar application of 0.2% Fe at both stages. Results also showed that maximum test weight was recorded when for soil application of Fe at 5.0 kg ha−1 which was statistically similar (p ≤ 0.05) to soil application of Fe at 2.5 kg ha−1 followed by foliar application of Fe at 0.2%. The minimum value of test weight was recorded for the control treatment. As compared with control, Fe application either by the soil or foliar spray enhanced grain yield during both years, however, there was a non-significant difference among the treatments. The minimum grain yield was recorded for the control treatment during both study years. Foliar application of 0.2% Fe at flowering initiation recorded maximum grain Fe content followed by foliar spray of 0.2% Fe one week after flowering initiation, soil application of Fe at 5.0 and 2.5 kg ha−1 (Fig. 3). The minimum Fe content was recorded for the control treatment during both study years. Furthermore, as shown in Table 3A, a correlation also exists between the collected parameters with grain yield; however, there was a non-significant correlation found with grain Fe contents.
Figure 3: Effect of iron (Fe) application rates on Fe contents in the grains of mungbean. T1, control; T2, soil application of Fe at 2.5 kg ha−1; T3, soil application of Fe at 5.0 kg ha−1; T4, foliar application of Fe at 0.2% at flowering initiation (FI) stage; T5, foliar application of Fe at 0.3% at one week after FI. The values are means ± SE, n = 3. Significant differences are shown by lowercase letters (at p ≤ 0.05)
3.3 Effects of B Application on Performance of Mungbean
Application of B significantly influenced the growth and yield traits in mungbean except for plant height during both years and the number of primary branches in 2019 (Table 4). In 2020, the number of primary branches was statistically similar among soil applied application of B at 0.55 and 1.1 kg ha−1 and foliar application of B at 0.1% solution at flowering initiation and one week after flowering initiation, while minimum values were recorded for the control. Soil application of B at 1.1 and 0.55 kg ha−1 produced higher but statistically similar pods per plant and pod length during both study years followed by the foliar application at 0.1% while the minimum value was recorded for the control treatment. In 2019, maximum seeds per pod were recorded when B was applied to the soil application at 1.1 kg ha−1, however, the values were statistically similar with soil-applied B at 0.55 kg ha−1 and foliar application at 0.1%. During the second year of study, maximum and statistically similar seeds per pod were recorded for the foliar spray of B at 0.1% at flowering initiation and one week after flowering initiation and soil application of B at 0.55 and 1.1 kg ha−1, while the minimum was recorded for the control treatment. Maximum test weight and grain yield were recorded for soil application of B at 1.1 kg ha−1 which were statistically at par with soil application of B at 0.55 kg ha−1, followed by foliar application of 0.1% B at flowering initiation and one week after flowering initiation. The minimum values of these traits were recorded for the control treatment during both years of study. Maximum grain B concentration was recorded for foliar application of 0.1% B at flowering initiation followed by its spray with the same concentration one week after flowering initiation and soil application of B at 0.55 and 1.1 kg ha−1. The minimum grain B concentration was recorded for the control treatment without B application (Fig. 4). A correlation exists between grain yield and other collected parameters but not with grain B contents (Table 4A).
Figure 4: Effect of boron (B) application rates on B contents in the grains of mungbean. T1, control; T2, soil application of B at 0.55 kg ha−1; T3, soil application of B at 1.10 kg ha−1; T4, foliar application B at 0.1% at flowering initiation (FI) stage; T5, foliar application of B at 0.1% at one week after FI. The values are means ± SE, n = 3. Significant differences are showed by lowercase letters (at p ≤ 0.05)
Means not sharing a letter in common differ significantly at 5% probability level.
The application of macro and micro-nutrient is essential for all field crops for better yield and quality [54]. Micronutrients (Fe, B, Zn and Se) [55] not only play a significant role in crop growth and development but also stimulate the human immune system. In our experiment, the application of Zn either through soil or foliar, significantly improved primary branches, plant height, pods per plant, pod length, seeds per pod, test weight and grain yield of mungbean. A positive correlation was also recorded among the recorded traits. However, soil application enhanced all these parameters more than foliar application. This increase might be attributed to the role of Zn in the synthesis of protein, lipids, carbohydrates, nucleic acid, nitrogen metabolism, cell division, photosynthesis [24], and all other metabolic processes of cells [56,57]. Better growth and yield of mungbean might also be attributed to the participation of Zn in several physiological processes during crop development [58], resistance to abiotic stress [59], nitrogen use efficiency [60], photosynthesis and protein synthesis [61]. The presence of Zn in cell triggers several enzymes involved in cell processes which ultimately enhance crop growth [62–64]. Zn plays a key role in auxin production which is involved in the enhancement of quantity and size of cell, resulting in taller plant height [65]. It has been reported by several scientists that the application of Zn at different levels enhanced the grain yield of mungbean [46,66–69]. Usman et al. [70] reported that Zn-fertilization enhanced grain and biological yield by enhancing the number of grains per pod and test weight of mungbean.
The increase in grain Zn concentration might be attributed to the remobilization of Zn reserves from vegetative parts to the grain [71]. Chen et al. [72] reported the increase in grain Zn concentration due to an increase in source Zn concentration through the soil and foliar application of Zn. Foliar application is an agronomic practice which is used for rapid biofortification of micronutrients in grains. Scientists believed that agronomic biofortification of Zn in wheat was more rapidly achieved than genetic or breeding biofortification [72,73]. Our study which indicates that Zn concentration of mungbean grains was significantly enhanced by the application of Zn mostly with foliar application of Zn than with soil-applied Zn are further supported by above-mentioned facts related to Zn. Scientists have reported the role of Fe in the respiratory electron transport chain (ETC), cell wall metabolism, photosynthesis and oxidative stress tolerance [74,75]. Fe being part of many enzymes is involved in the activation of a number of enzymes, cytochrome (involved in ETC), chlorophyll synthesis and chloroplast structure [76]. Micronutrients, especially Fe has great importance in photosynthesis and respiration as it is involved in several enzymatic activities and chlorophyll [21,77]. Moreover, it has been reported that Fe contributes in the synthesis of chlorophyll [78] and several plant growth regulators. An increase in plant height of mungbean has been reported by Khoulenjani et al. [79], however, an increase in test weight, number of grains, grain and straw yield and grain Fe concentration in wheat has been reported by Ziaeian et al. [80]. An increase in grain Fe iron concentration might also be attributed to the availability of Fe at the reproductive stage of mungbean due to foliar application. It has been previously observed that Fe application significantly enhanced the grain Fe concentration in wheat and groundnut [81,82]. Our results indicate that soil and foliar application of Fe significantly enhanced primary branches, plant height, pods per plant, pod length, seeds per pod, test weight, grain yield by soil application and grain Fe concentration by foliar application either in both years of study or in one are further supported by the above-mentioned roles of Fe in crops.
Boron plays a key role in cell wall formation, carbohydrate, Indoleacetic acid (IAA) and Ribonucleic acid (RNA) metabolism, membrane integrity, calcium uptake, translocation of sugars, flowering, pollination and ultimately growth [83–85]. The increase in primary branches, pods per plant and pod length is attributed to the role of B in cell elongation and maturation, protein synthesis, development of meristematic tissues and eventually plant growth and yield [86–90]. Jing et al. [91] reported that the application of B improves plant dry matter and pods per plant in groundnut. The foliar application of B at the flowering stage enhances the concentration of B in the source which ultimately is remobilized to sink. An increase in micronutrient concentration in flag leaf and grains was previously verified by Zeidan et al. [92] and Gomaa et al. [93]. The results of our study which indicate that application of B improved pods per plant, pod length, seeds per pod, test weight, grain yield and grain B concentration by soil application and foliar application of B, are further supported by the above-mentioned facts related to B.
This study is unique of its kind as it has addressed the vitally important issue of hidden hunger and presented a pragmatic solution for combating it as well. It was concluded that soil application of Zn, Fe and B is beneficial for better growth and yield of mungbean. However, foliar application of 0.3% Zn, 0.2% Fe and 0.1% B solution (w/v) at flowering initiation of mungbean proved to be more beneficial in enhancing the yield and grain contents of these micronutrients. A strong correlation of all parameters was found with grain yield however there was no correlation of grain Zn, Fe and B contents with all other collected parameters.
Authorship: Study conception and design: Muhammad Zafar, Siraj Ahmed, Muhammad Kashif Munir; data collection: Nawal Zafar, Muhammad Saqib; analysis and interpretation of results: Siraj Ahmed, Sadam Hussain, Aleem Sarwar, Saba Iqbal; Formal analysis: Aneela Gulnaz, Baber Ali, Mohammad Khalid Al-Sadoon; draft manuscript preparation: Siraj Ahmed, Muhammad Saqib; Funding acquisition: Aneela Gulnaz, Baber Ali, Mohammad Khalid Al-Sadoon. All authors reviewed the results and approved the final version of the manuscript.
Acknowledgement: The authors would like to extend their sincere appreciation to the Researchers Supporting Project No. (RSP2023R410), King Saud University, Riyadh, Saudi Arabia. The authors also would like to thank Punjab Agricultural Research Board, Pakistan for funding the Research Project PARB No. 904.
Funding Statement: This research work is supported by Researchers Supporting Project No. (RSP2023R410), King Saud University, Riyadh, Saudi Arabia.
Conflicts of Interest: The authors declare that they have no conflicts of interest to report regarding the present study.
References
1. Singh, R., van Heusden, A. W., Kumar, R., Visser, R. G. (2018). Genetic variation and correlation studies between micronutrient (Fe and Znprotein content and yield attributing traits in mungbean (Vigna radiata L.). Legume Research–An International Journal, 41(2), 167–174. [Google Scholar]
2. Ahmed, F., Haraguchi, T., Hirota, O., Rahman, M. A. (2000). Growth analysis, yield, and canopy structure in maize-mungbean intercropping. Bulliten of Institute of Tropical Agriculture, Kyushu University, 23, 61–69. [Google Scholar]
3. Haider, M. U., Hussain, M., Farooq, M., Ul-Allah, S., Ansari, M. J. et al. (2021). Zinc biofortification potential of diverse mungbean [Vigna radiata (L.) Wilczek] genotypes under field conditions. PLoS One, 16(6), e0253085. [Google Scholar]
4. GoP (2021). Pakistan economic survey: Agriculture. Islamabad, Pakistan. https://www.finance.gov.pk/survey/chapter_20/02_Agriculture.pdf. [Google Scholar]
5. Ahmad, M., Ishaq, M., Shah, W. A., Adnan, M., Fahad, S. et al. (2022). Managing phosphorus availability from organic and inorganic sources for optimum wheat production in calcareous soils. Sustainability, 14(13), 7669. DOI 10.3390/su14137669. [Google Scholar] [CrossRef]
6. Haider, M. W., Nafees, M., Ahmad, I., Ali, B., Maryam et al. (2022). Postharvest dormancy-related changes of endogenous hormones in relation to different dormancy-breaking methods of potato (Solanum tuberosum L.) tubers. Frontiers in Plant Science, 13, 945256. DOI 10.3389/fpls.2022.945256. [Google Scholar] [CrossRef]
7. Nasiri, Y., Zehtab-Salmasi, S., Nasrullahzadeh, S., Najafi, N., Ghassemi-Golezani, K. (2010). Effects of foliar application of micronutrients (Fe and Zn) on flower yield and essential oil of chamomile (Matricaria chamomilla L.). Journal of Medicinal Plants Research, 4(17), 1733–1737. [Google Scholar]
8. Shenkin, A. (2006). Micronutrients in health and disease. Postgraduate Medical Journal, 82(971), 559–567. [Google Scholar]
9. FAO (2017) The state of food security and nutrition in the world. 2nd edition. Rome (ItalyFood and Agriculture Organization. [Google Scholar]
10. Gibson, R. S. (2012). Zinc deficiency and human health: Etiology, health consequences, and future solutions. Plant and Soil, 361(1), 291–299. [Google Scholar]
11. Stevens, G., Mascarenhas, M., Mathers, C. (2009). Global health risks: Progress and challenges. Bulletin of the World Health Organization, 87, 646. [Google Scholar]
12. Ministry of Health (2009). National health. In: Stepping towards better health. Islamabad, Pakistan: Ministry of Health. [Google Scholar]
13. Glahn, R. P., Noh, H. (2021). Redefining bean iron biofortification: A review of the evidence for moving to a high Fe bioavailability approach. Frontiers in Sustainable Food Systems, 5, 215. [Google Scholar]
14. Petry, N., Olofin, I., Hurrell, R. F., Boy, E., Wirth, J. P. et al. (2016). The proportion of anemia associated with iron deficiency in low, medium, and high human development index countries: A systematic analysis of national surveys. Nutrients, 8(11), 693. DOI 10.3390/nu8110693. [Google Scholar] [CrossRef]
15. Hummel, M., Talsma, E. F., Taleon, V., Londoño, L., Brychkova, G. et al. (2020). Iron, zinc and phytic acid retention of biofortified, low phytic acid, and conventional bean varieties when preparing common household recipes. Nutrients, 12(3), 658. DOI 10.3390/nu12030658. [Google Scholar] [CrossRef]
16. Cakmak, I. (2011). Zinc plays critical role in plant growth. http://www.zinc.org/crops/resourceserve/zinc_plays_critical_role_in_plant_growth. [Google Scholar]
17. Hussain, A., Zahir, Z. A., Asghar, H. N., Ahmad, M., Jamil, M. et al. (2018). Zinc solubilizing bacteria for zinc biofortification in cereals: A step toward sustainable nutritional security. In: Role of rhizospheric microbes in soil, pp. 203–227. Springer Nature Singapore Pte, Ltd. [Google Scholar]
18. Pathak, G. C., Gupta, B., Pandey, N. (2012). Improving reproductive efficiency of chickpea by foliar application of zinc. Brazilian Journal of Plant Physiology, 24(3), 173–180. DOI 10.1590/S1677-04202012000300004. [Google Scholar] [CrossRef]
19. Broadley, M. R., White, P. J., Hammond, J. P., Zelko, I., Lux, A. (2007). Zinc in plants. New Phytologist, 173(4), 677–702. DOI 10.1111/j.1469-8137.2007.01996.x. [Google Scholar] [CrossRef]
20. Alloway, B. J. (2009). Soil factors associated with zinc deficiency in crops and humans. Environmental Geochemistry and Health, 31(5), 537–548. DOI 10.1007/s10653-009-9255-4. [Google Scholar] [CrossRef]
21. Ali, B., Wang, X., Saleem, M. H., Azeem, M. A., Afridi, M. S. et al. (2022). Bacillus mycoides PM35 reinforces photosynthetic efficiency, antioxidant defense, expression of stress-responsive genes, and ameliorates the effects of salinity stress in maize. Life, 12(2), 219. DOI 10.3390/life12020219. [Google Scholar] [CrossRef]
22. Seher, B. A., Ozturk, L., Gokmen, O. O., Römheld, V., Cakmak, I. (2011). Effect of nitrogen on root release of phytosiderophores and root uptake of Fe(III)-phytosiderophore in Fe-deficient wheat plants. Physiologia Plantarum, 142(3), 287–296. [Google Scholar]
23. Niaz, A., Ranjha, A. M., Rahmatullah, A. H., Waqas, M. (2007). Boron status of soils as affected by different soil characteristics-pH, CaCO3, organic matter and clay contents. Pakistan Journal of Agricultural Sciences, 44(3), 428–435. [Google Scholar]
24. Saini, A., Manuja, S., Kumar, S., Hafeez, A., Ali, B. et al. (2022). Impact of cultivation practices and varieties on productivity, profitability, and nutrient uptake of rice (Oryza sativa L.) and wheat (Triticum aestivum L.) cropping system in India. Agriculture, 12(10), 1678. DOI 10.3390/agriculture12101678. [Google Scholar] [CrossRef]
25. Naz, R., Khan, M. K., Hafeez, A., Fazil, M., Khan, M. N. et al. (2024). Assessment of phytoremediation potential of native plant species naturally growing in a heavy metal-polluted industrial soils. Brazilian Journal of Biology, 84(1–4), e264473. DOI 10.1590/1519-6984.264473. [Google Scholar] [CrossRef]
26. Rashid, A., Ryan, J. (2008). Micronutrient constraints to crop production in the Near East. In: Brian, J., Alloway, B. J. (Eds.Micronutrient deficiencies in global crop production, pp. 149–180. Netherlands, Dordrecht: Springer Dordrecht. [Google Scholar]
27. El Habbasha, S., Mohamed, M. H., El-Lateef, E. A., Mekki, B. B., Ibrahim, M. E. (2013). Effect of combined zinc and nitrogen on yield, chemical constituents and nitrogen use efficiency of some chickpea cultivars under sandy soil conditions. World Journal of Agricultural Sciences, 9(4), 354–360. [Google Scholar]
28. Chasapis, C. T., Loutsidou, A. C., Spiliopoulou, C. A., Stefanidou, M. E. (2012). Zinc and human health: An update. Archives of Toxicology, 86(4), 521–534. DOI 10.1007/s00204-011-0775-1. [Google Scholar] [CrossRef]
29. Thakur, A., Singh, S., Dulta, K., Singh, N., Ali, B. et al. (2022). Nutritional evaluation, phytochemical makeup, and antibacterial and antioxidant properties of wild plants utilized as food by the Gaddis, a tribe in the Western Himalayas. Frontiers in Agronomy, 4, 1010309. DOI 10.3389/fagro.2022.1010309. [Google Scholar] [CrossRef]
30. Black, R. E., Allen, L. H., Bhutta, Z. A., Caulfield, L. E., Onis, M. D. et al. (2008). Maternal and child undernutrition: Global and regional exposures and health consequences. Lancet, 371(9608), 243–260. DOI 10.1016/S0140-6736(07)61690-0. [Google Scholar] [CrossRef]
31. Cakmak, I. (2008). Enrichment of cereal grains with zinc: Agronomic or genetic biofortification. Plant and Soil, 302(1), 1–17. DOI 10.1007/s11104-007-9466-3. [Google Scholar] [CrossRef]
32. Rehman, A., Farooq, M., Nawaz, A., Ahmad, R. (2014). Influence of boron nutrition on the rice productivity, kernel quality and biofortification in different production systems. Field Crops Research, 169, 123–131. [Google Scholar]
33. Food and Nutrition Board, Institute of Medicine, National Academy of Sciences (2001). Dietary reference intakes for vitamin a, vitamin k, arsenic, boron, chromium, copper, iodine, iron, manganese, molybdenum, nickel, silicon, vanadium, and zinc. Washington DC: National Academy Press. [Google Scholar]
34. Aggett, P. J. (2012). Present knowledge in nutrition. In: Erdman, J. W., Macdonald, I. A., Zeisel, S. H. (Eds.Wiley-blackwell, 10th edition. pp. 506–520. Washington DC: John Wiley & Sons. [Google Scholar]
35. Moretti, D., Zimmermann, M. B., Muthayya, S., Thankachan, P., Lee, T. C. et al. (2006). Extruded rice fortified with micronized ground ferric pyrophosphate reduces iron deficiency in Indian schoolchildren: A double-blind randomized controlled trial. The American Journal of Clinical Nutrition, 84(4), 822–829. [Google Scholar]
36. Stevens, G. A., Finucane, M. M., De-Regil, L. M., Paciorek, C. J., Flaxman, S. R. et al. (2013). Nutrition Impact Model Study Group. Global, regional, and national trends in Haemoglobin concentration and prevalence of total and severe anaemia in children and pregnant and non-pregnant women for 1995–2011: A systematic analysis of population-representative data. The Lancet Global Health, 1(1), 16–25. [Google Scholar]
37. Białek, M., Czauderna, M., Krajewska, K. A., Przybylski, W. (2019). Selected physiological effects of boron compounds for animals and humans. A review. Journal of Animal and Feed Sciences, 28(4), 307–320. [Google Scholar]
38. Sizmaz, O., Koksal, B., Yildiz, G. (2017). Rumen microbial fermentation, protozoan abundance and boron availability in yearling rams fed diets with different boron concentrations. Journal of Animal and Feed Sciences, 26(1), 59–64. [Google Scholar]
39. Hunt, C. (2006). Dietary boron: Progress in establishing essential roles in human and animal physiology. Proceedings of 3rd International Boron Symposium, pp. 3–10. Ankara, Turkey. [Google Scholar]
40. Scorei, R. I., Rotaru, P. (2011). Calcium fructoborate—potential anti-inflammatory agent. Biological Trace Element, 143(3), 1223–1238. DOI 10.1007/s12011-011-8972-6. [Google Scholar] [CrossRef]
41. Horiguchi, H., Satom, T., Kumada, H., Yamamoto, T., Sakae, T. (2015). Estimation of relative biological effectiveness for boron neutron capture therapy using the PHITS code coupled with a microdosimetric kinetic model. Journal of Radiation Research, 56(2), 382–390. DOI 10.1093/jrr/rru109. [Google Scholar] [CrossRef]
42. De-Valença, A. W., Bake, A., Brouwer, I. D., Giller, K. E. (2017). Agronomic biofortification of crops to fight hidden hunger in Sub-Saharan Africa. Global Food Security, 12, 8–14. DOI 10.1016/j.gfs.2016.12.001. [Google Scholar] [CrossRef]
43. Yasmin, A., Mannan, M. A., Sarker, U., Dola, D. B., Higuchi, H. et al. (2022). Foliar application of seaweed extracts enhances yield and drought tolerance of soybean. Frontiers in Plant Science, 13, 992880. [Google Scholar]
44. El-Dahshouri, M. F. (2017). Effect of zinc foliar application at different physiological growth stages on yield and quality of wheat under sandy soil conditions. Agricultural Engineering International: CIGR Journal, 193–200. [Google Scholar]
45. Hussan, M. U., Hafeez, M. B., Saleem, M. F., Khan, S., Hussain, S. et al. (2021). Impact of soil applied humic acid, zinc and boron supplementation on the growth, yield and zinc translocation in winter wheat. Asian Journal of Agriculture and Biology, 2022(1), 202102080. DOI 10.35495/ajab.2021.02.080. [Google Scholar] [CrossRef]
46. PABRA (2017). PABRA Annual Report 2016/2017. 20th Anniversary Special Edition. Kenya, Nairobi: Pan-Africa Bean Research Alliance-PABRA. [Google Scholar]
47. Haas, J. D., Luna, S. V., Lung’aho, M. G., Wenger, M. J., Murray-Kolb, L. E. et al. (2016). Consuming iron biofortified beans increases iron status in Rwandan women after 128 days in a randomized controlled feeding trial. The Journal of Nutrition, 146(8), 1586–1592. [Google Scholar]
48. Bouis, H. E., Welch, R. M. (2010). Biofortification—A sustainable agricultural strategy for reducing micronutrient malnutrition in the global south. Crop Science, 50(1), S-20–S-32. [Google Scholar]
49. Chapman, H. D., Pratt, P. F. (1961). Methods of analysis for soils, plants and water. Berkeley, CA, USA: University California. [Google Scholar]
50. Bingham, F. T., Boron (1982). Methods of soil analysis, Part2: Chemical and mineralogical properties. In: Page, A. L. (Ed.American society of agronomy, pp. 431–448. Madison, WI, USA: Scientific Research Publishing Inc. [Google Scholar]
51. Rashid, A. (1986). Mapping zinc fertility of soils using indicator plants and soil analyses. USA, Honolulu: University of Hawai’i at Manoa. [Google Scholar]
52. Estefan, G., Sommer, E., Ryan, J. (2013Methods of soil, plant and water analysis: A manual for the west Asia and North Africa region. 3rd edition. Beirut, Lebanon: International Center for Agricultural Research in the Dry Areas (ICARDA). [Google Scholar]
53. Steel, R. G. D., Torrie, J. H., Dickey, D. A. (1997). Principles and procedures of statistics: A biometrical approach. 3rd edition. New York: McGraw Hill Book Co. Inc. [Google Scholar]
54. Faryal, S., Ullah, R., Khan, M. N., Ali, B., Hafeez, A. et al. (2022). Thiourea-capped nanoapatites amplify osmotic stress tolerance in Zea mays l. by conserving photosynthetic pigments, osmolytes biosynthesis and antioxidant biosystems. Molecules, 27(18), 5744. DOI 10.3390/molecules27185744. [Google Scholar] [CrossRef]
55. din Umar, U., Ahmed, N., Zafar, M. Z., Rehman, A., Naqvi, S. A. H. et al. (2022). Micronutrients foliar and drench application mitigate mango sudden decline disorder and impact fruit yield. Agronomy, 12, 2449. [Google Scholar]
56. Salam, A., Afridi, M. S., Javed, M. A., Saleem, A., Hafeez, A. et al. (2022). Nano-priming against abiotic stress: A way forward towards sustainable agriculture. Sustainability, 14, 14880. [Google Scholar]
57. Ali, S., Ullah, S., Khan, M. N., Khan, W. M., Razak, S. A. et al. (2022). The effects of osmosis and thermo-priming on salinity stress tolerance in Vigna radiata L. Sustainability, 14, 12924. [Google Scholar]
58. Ma, J., Saleem, M. H., Ali, B., Rasheed, R., Ashraf, M. A. et al. (2022). Impact of foliar application of syringic acid on tomato (Solanum lycopersicum L.) under heavy metal stress-insights into nutrient uptake, redox homeostasis, oxidative stress, and antioxidant defense. Frontiers in Plant Science, 13, 950120. DOI 10.3389/fpls.2022.950120. [Google Scholar] [CrossRef]
59. Ma, J., Saleem, M. H., Yasin, G., Mumtaz, S., Qureshi, F. F. et al. (2022). Individual and combinatorial effects of SNP and NaHS on morpho-physio-biochemical attributes and phytoextraction of chromium through Cr-stressed spinach (Spinacia oleracea L.). Frontiers in Plant Science, 13, 973740. DOI 10.3389/fpls.2022.973740. [Google Scholar] [CrossRef]
60. Wang, K., Ding, Y., Cai, C., Chen, Z., Zhu, C. (2019). The role of C2H2 zinc finger proteins in plant responses to abiotic stresses. Physiologia Plantarum, 165(4), 690–700. [Google Scholar]
61. Mehmood, S., Khatoon, Z., Amna, Ahmad, I., Muneer, M. A. et al. (2021). Bacillus sp. PM31 harboring various plant growth-promoting activities regulates Fusarium dry rot and wilt tolerance in potato. Archives of Agronomy and Soil Science, 2021, 1–15. [Google Scholar]
62. Wahab, A., Abdi, G., Saleem, M. H., Ali, B., Ullah, S. et al. (2022). Physio-biochemical and phyto-hormonal responses to alleviate the adverse effects of drought stress: A comprehensive review. Plants, 11, 1620. DOI 10.3390/plants11131620. [Google Scholar] [CrossRef]
63. Zainab, N., Amna, Khan, A. A., Azeem, M. A., Ali, B. et al. (2021). PGPR-mediated plant growth attributes and metal extraction ability of Sesbania sesban L. Agronomy, 11(9), 1820. DOI 10.3390/agronomy11091820. [Google Scholar] [CrossRef]
64. Nawaz, H., Ali, A., Saleem, M. H., Ameer, A., Hafeez, A. et al. (2022). Comparative effectiveness of EDTA and citric acid assisted phytoremediation of Ni contaminated soil by using canola (Brassica napus). Brazilian Journal of Biology, 82, e261785. [Google Scholar]
65. Dashadi, M., Hossein, A., Radjabi, R., Babainejad, T. (2013). Investigation of effect different rates Phosphorus and Zinc fertilizers on two cultivars Lentil (Gachsaran and Flip92-12L) in irrigation complement condition. International Journal of Agriculture and Crop Sciences, 5, 1–5. [Google Scholar]
66. Haider, M. U., Hussain, M., Farooq, M., Nawaz, A. (2018). Soil application of zinc improves the growth, yield and grain zinc biofortification of mungbean. Soil and Environment, 37(2), 123–128. [Google Scholar]
67. Haider, M. U., Hussain, M., Farooq, M., Nawaz, A. (2020a). Optimizing zinc seed priming for improving the growth, yield and grain biofortification of mungbean (Vigna radiata (L.) wilczek). Journal of Plant Nutrition, 43(10), 1438–1446. [Google Scholar]
68. Haider, M. U., Hussain, M., Farooq, M., Nawaz, A. (2020b). Zinc nutrition for improving the productivity and grain biofortification of mungbean. Journal of Soil Science and Plant Nutrition, 20(3), 1321–1335. [Google Scholar]
69. Haider, M. U., Hussain, M., Farooq, M. (2019). Optimizing zinc seed coating treatments for improving growth, productivity and grain biofortification of mungbean. Soil & Environment, 38(1), 97–102. [Google Scholar]
70. Usman, M., Tahir, M., Majeed, M. A. (2014). Effect of zinc sulphate as soil application and seed treatment on green gram (Vigna radiata L.). Pakistan Journal of Life and Social Sciences, 12(2), 87–91. [Google Scholar]
71. Rose, T., Bowden, B. (2013). Matching soil nutrient supply and crop demand during the growing season; Improving water and nutrient use efficiency in food production systems, pp. 93–103. Ames: Wiley. [Google Scholar]
72. Chen, X. P., Zhang, Y. Q., Tong, Y. P., Xue, Y. F., Liu, D. Y. et al. (2017). Harvesting more grain zinc of wheat for human health. Scientific Reports, 7(1), 1–8. [Google Scholar]
73. Cakmak, I., Kutman, U. B. (2018). Agronomic biofortification of cereals with zinc: A review. European Journal of Soil Science, 69(1), 172–180. [Google Scholar]
74. Amna, A. B., Azeem, M. A., Qayyum, A., Mustafa, G., Ahmad, M. A. et al. (2021). Bio-fabricated silver nanoparticles: A sustainable approach for augmentation of plant growth and pathogen control. In: Sustainable agriculture reviews, vol. 53, pp. 345–371. Cham: Springer. [Google Scholar]
75. Dola, D. B., Mannan, M. A., Sarker, U., Mamun, M. A. A., Islam, T. et al. (2022). Nano-iron oxide accelerates growth, yield, and quality of Glycine max seed in water deficits. Frontiers in Plant Science, 13, 992535. DOI 10.3389/fpls.2022.992535. [Google Scholar] [CrossRef]
76. Farooq, T. H., Rafay, M., Basit, H., Shakoor, A., Shabbir, R. et al. (2022). Morpho-physiological growth performance and phytoremediation capabilities of selected xerophyte grass species toward Cr and Pb stress. Frontiers in Plant Science, 13, 997120. DOI 10.3389/fpls.2022.997120. [Google Scholar] [CrossRef]
77. Ali, B., Wang, X., Saleem, M. H., Hafeez, A., Afridi, M. S. et al. (2022). PGPR-mediated salt tolerance in maize by modulating plant physiology, antioxidant defense, compatible solutes accumulation and bio-surfactant producing genes. Plants, 11(3), 345. DOI 10.3390/plants11030345. [Google Scholar] [CrossRef]
78. Saleem, K., Asghar, M. A., Saleem, M. H., Raza, A., Kocsy, G. et al. (2022). Chrysotile-asbestos-induced damage in Panicum virgatum and Phleum pretense species and its alleviation by organic-soil amendment. Sustainability, 14(17), 10824. DOI 10.3390/su141710824. [Google Scholar] [CrossRef]
79. Khoulenjani, M. B., Salamati, M. S. (2012). Morphological reaction and yield of Nigella sativa L. to Fe and Zn. African Journal of Agricultural Research, 7(15), 2359–2362. [Google Scholar]
80. Ziaeian, A. H., Malakouti, M. J. (2001). Effect of micronutrient application on wheat production in calcareous soils. Second National Conference on Optimum Utilization of Chemical Fertilizers and Pesticide in agriculture, Karaj, Iran. [Google Scholar]
81. Pahlavan-Rad, M. R., Pessarakli, M. (2009). Response of wheat plants to zinc, iron, and manganese applications and uptake and concentration of zinc, iron, and manganese in wheat grains. Communications in Soil Science and Plant Analysis, 40(7–8),1322–1332. [Google Scholar]
82. Patel, M. S., Suthar, D. M., Kanzaria, M. V. (1993). Effect of foliar application of iron and sulphur in curing chlorosis in groundnut. Journal of the Indian Society of Soil Science, 41(1), 103–105. [Google Scholar]
83. Afridi, M. S., Javed, M. A., Ali, S., de Medeiros, F. H. V., Ali, B. et al. (2022). New opportunities in plant microbiome engineering for increasing agricultural sustainability under stressful conditions. Frontiers in Plant Science, 13, 899464. DOI 10.3389/fpls.2022.899464. [Google Scholar] [CrossRef]
84. Ali, B., Hafeez, A., Javed, M. A., Afridi, M. S., Abbasi, H. A. (2022). Role of endophytic bacteria in salinity stress amelioration by physiological and molecular mechanisms of defense: A comprehensive review. South African Journal of Botany, 151, 33–46. DOI 10.1016/j.sajb.2022.09. [Google Scholar] [CrossRef]
85. Ali, B., Hafeez, A., Ahmad, S., Javed, M. A., Sumaira et al. (2022). Bacillus thuringiensis PM25 ameliorates oxidative damage of salinity stress in maize via regulating growth, leaf pigments, antioxidant defense system, and stress responsive gene expression. Frontiers in Plant Science, 13, 921668. DOI 10.3389/fpls.2022.921668. [Google Scholar] [CrossRef]
86. Akram, N. A., Saleem, M. H., Shafiq, S., Naz, H., Farid-ul-Haq, M. et al. (2022). Phytoextracts as crop biostimulants and natural protective agents—A critical review. Sustainability, 14, 14498. [Google Scholar]
87. Bibi, S., Ullah, S., Hafeez, A., Khan, M. N., Javed, M. A. et al. (2024). Exogenous Ca/Mg quotient reduces the inhibitory effects of PEG induced osmotic stress on Avena sativa L. Brazilian Journal of Biology, 84(2), e264642. DOI 10.1590/1519-6984.264642. [Google Scholar] [CrossRef]
88. Fahad, S., Chavan, S. B., Chichaghare, A. R., Uthappa, A. R., Kumar, M. et al. (2022). Agroforestry systems for soil health improvement and maintenance. Sustainability, 14(22), 14877. DOI 10.3390/su142214877. [Google Scholar] [CrossRef]
89. Saeed, S., Ullah, A., Ullah, S., Noor, J., Ali, B. et al. (2022). Validating the impact of water potential and temperature on seed germination of wheat (Triticum aestivum L.) via Hydrothermal Time Model. Life, 12, 983. [Google Scholar]
90. Hussain, S. Q., Rasheed, M., Saleem, M. H., Ahmed, Z. I., Hafeez, A. et al. (2023). Salt tolerance in maize with melatonin priming to achieve sustainability in yield on salt affected soils. Pakistan Journal of Botany, 55(1). DOI 10.30848/PJB2023-1(27). [Google Scholar] [CrossRef]
91. Jing, R. F., Zhang, Q. G., Han, L. F., Zhang, F. S., Wei, X. Q. (1994). Effect of boric fertilizer on peanut absorption of boron and nitrogen. Soils, 26, 83–86. [Google Scholar]
92. Zeidan, M. S., Mohamed, M. F., Hamouda, H. A. (2010). Effect of foliar fertilization of Fe, Mn and Zn on wheat yield and quality in low sandy soils fertility. World Journal of Agricultural Sciences, 6(6), 696–699. [Google Scholar]
93. Gomaa, M. A., Radwan, F. I., Kandil, E. E., El-Zweek, S. M. A. (2015). Effect of some macro and micronutrients application methods on productivity and quality of wheat (Triticum aestivum L.). Middle East Journal of Agricultural Research, 4(1), 1–10. [Google Scholar]
Cite This Article
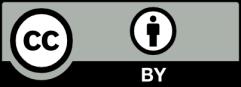