Open Access
ARTICLE
High-Value-Added Utilization of Turpentine: Screening of Anti-Influenza Virus Agents from β-Pinene Derivatives
1 East China Woody Fragrance and Flavor Engineering Research Center of National Forestry and Grassland Administration, Camphor Engineering Research Center of National Forestry and Grassland Administration, College of Forestry, Jiangxi Agricultural University, Nanchang, 330045, China
2 Institute of Chemical Industry of Forestry Products, China Academy of Forestry, National Engineering Laboratory for Biomass Chemical Utilization, Key and Open Laboratory of Forest Chemical Engineering, State Forestry Administration, Key Laboratory of Biomass Energy and Material, Jiangsu Province, Nanjing, 210042, China
3 College of Chemical Engineering, Huaqiao University, Xiamen, 361021, China
* Corresponding Authors: Zongde Wang. Email: ; Shengliang Liao. Email:
# These authors contributed equally to this work
(This article belongs to the Special Issue: Renewable Material from Agricultural Waste and By-Product and Its Applications)
Journal of Renewable Materials 2024, 12(1), 45-56. https://doi.org/10.32604/jrm.2023.031089
Received 13 May 2023; Accepted 28 July 2023; Issue published 23 January 2024
Abstract
Turpentine is a renewable and resourceful forest product. The deep processing and utilization of turpentine, particularly its primary component β-pinene, has garnered widespread attention. This study aimed to synthesize 40 derivatives of β-pinene, including nopinone, 3-cyanopyridines of nopinone, myrtanyl acid, myrtanyl acylthioureas, and myrtanyl amides. We assessed the antiviral activities of these β-pinene derivatives against influenza virus A/Puerto Rico/8/34 (H1N1) using the 3-(4,5-dimetylthiazol-2-yl)-2,5-diphenyltetrazolium bromide method. The β-pinene derivatives were used before and after cellular infection with the influenza virus to evaluate their preventive and therapeutic effects against the H1N1 virus. The results showed that only compound 10o exhibited a preventive effect against the H1N1 virus with a half-maximal inhibitory concentration (IC50) value of 47.6 μmol/L. Among the compounds, 4e, 4i, and 4l demonstrated therapeutic effects against cellular infection, with compound 4e displaying the most potent therapeutic effect (IC50 = 17.5 μmol/L), comparable to the positive control ribavirin. These findings indicated that certain β-pinene derivatives exhibited in vitro antiviral activity against the H1N1 influenza A virus, warranting further investigation as potential anti-influenza agents.Graphical Abstract
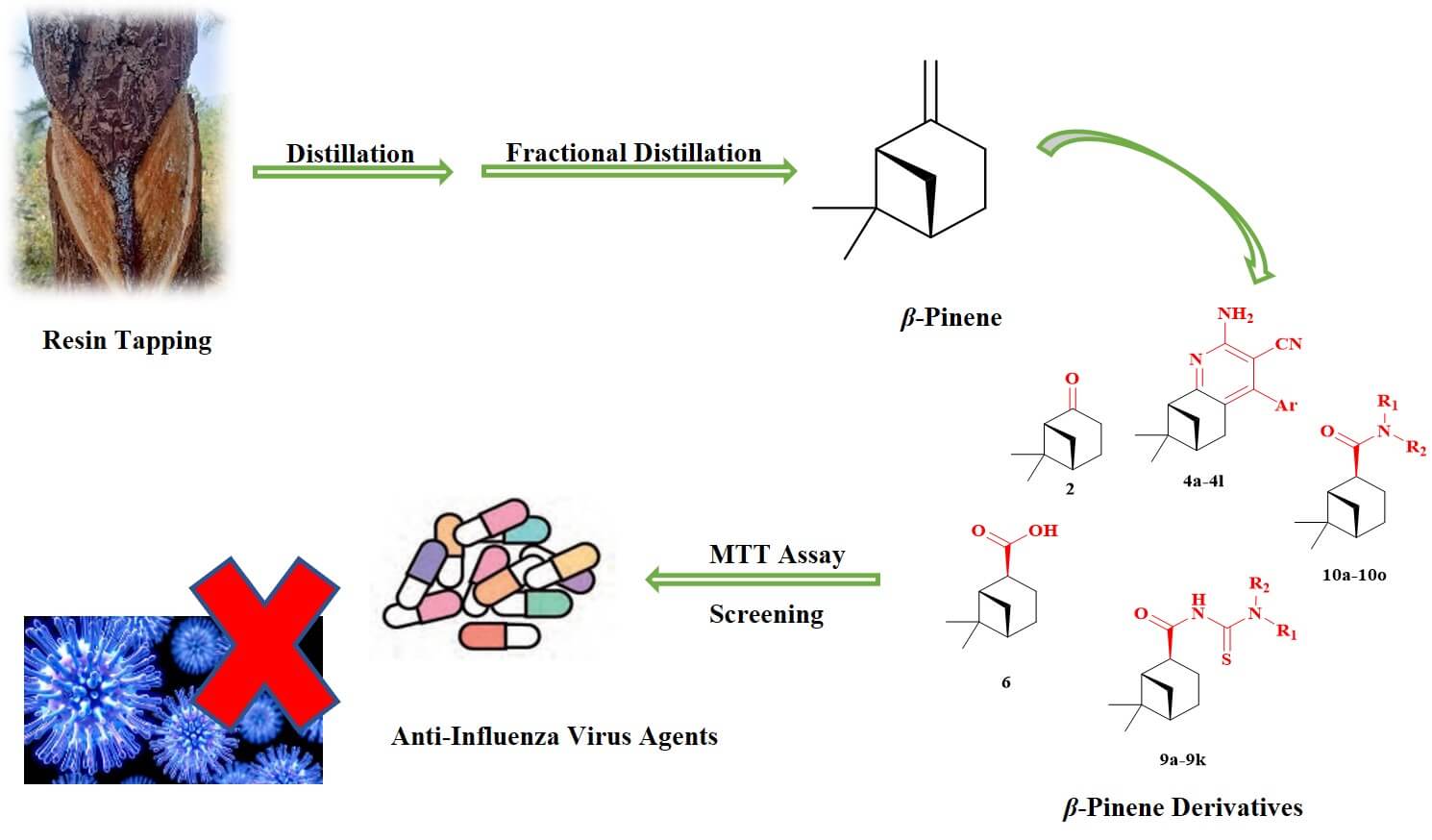
Keywords
Supplementary Material
Supplementary Material FileThe coronavirus disease 2019 pandemic, which began in early 2020, has had a profound global impact, resulting in widespread economic contraction, millions of confirmed cases, and a significant loss of lives [1]. Viruses have existed on Earth for hundreds of millions of years, and can cause various infectious diseases posing a threat to human life and health. Influenza is a relatively common acute respiratory infection caused by the influenza virus. Unlike the common cold, it exhibits more intense symptoms and is highly contagious, thus posing a higher risk of causing widespread epidemics. Tragically, seasonal influenza–associated respiratory illnesses claim numerous lives worldwide every year [2].
Influenza viruses are generally divided into three types: influenza A, influenza B, and, less commonly, influenza C. Among these, influenza A is the most infectious and pathogenic virus [3]. The “Spanish flu,” one of the most devastating pandemics in history, was caused by the influenza A (H1N1) virus, resulting in the death of approximately 50–100 million people, surpassing the casualties of World War I [4]. Notably, unlike many influenza strains primarily affecting specific populations, such as children, elderly, and immunocompromised individuals, influenza A (H1N1) viruses predominantly target young, healthy adults. Symptoms commonly include fever, cough, sore throat, and typical influenza manifestations such as headaches and generalized aches. Complications such as pneumonia, otitis media, and myocarditis may arise in severe cases. Influenza vaccines and conventional antiviral drugs are widely used for prevention and treatment in clinical practice. However, the emergence of a number of drug-resistant H1N1 virus strains necessitates the urgent need to develop new anti-influenza A (H1N1) virus drugs, so as to effectively combat the persistent threat posed by the H1N1 virus [5–7].
Plant essential oils offer numerous advantages including broad-spectrum antibacterial and antiviral activities, minimal toxic side effects, and affordability [8–11]. Turpentine, a natural plant essential oil, is abundantly available and represents one of the largest renewable resources globally. β-pinene is a significant component of turpentine and is classified as a monoterpene secondary metabolite characterized by its polycyclic, bridging ring and double bond structures. β-pinene can be synthesized into diverse derivatives with substantial added value through various chemical reactions such as oxidation and esterification. β-pinene derivatives exhibit various biological activities, including antimicrobial [12,13], herbicidal [14], insecticidal [15], repelling [16], anticoagulant [17], and anticancer activities [18]. However, only a few studies have reported on the potential of β-pinene derivatives against the influenza A (H1N1) virus.
Therefore, this study screened 40 β-pinene derivatives to evaluate their inhibitory activity against the A/Puerto Rico/8/34 (H1N1) virus and analyzed the preliminary structure–activity relationship between the molecular structure and antiviral activity of these compounds. The findings of this study provided an experimental basis for further research and might contribute to the development of effective drugs against influenza virus infections. Additionally, these results might enhance the perceived value of β-pinene as a potential anti-influenza virus drug in the pharmaceutical field.
2.1 Preparation of β-Pinene Derivatives
2.1.1 Materials and Structural Characterization Techniques
(-)-β-Pinene was purchased from Jiangxi Jishui Hongda Natural Spice Co., Ltd. (China), and other reagents were purchased from Aladdin Industrial Corporation (Shanghai, China) and Macklin (Shanghai, China) at analytical grade. All commercial reagents were used without further purification. β-Pinene derivatives were confirmed by 1H nuclear magnetic resonance (NMR), 13C NMR, Fourier transform infrared spectroscopy, and mass spectroscopy (MS), as described in the Supplementary Materials.
2.1.2 Synthesis of β-Pinene Derivatives
A total of 40 β-pinene derivatives, including nopinone (compound 2), 12 3-cyanopyridines of nopinone (compounds 4a–4l), myrtanyl acid (compound 6), 11 myrtanyl acylthioureas (compound 9a–9k), and 15 myrtanyl amides (compounds 10a–10o), were synthesized following the method described in previous studies [19,20]. Their synthetic routes are depicted in Fig. 1.
Figure 1: Synthetic routes of the β-pinene derivatives
2.2 Evaluation of Biological Activity
The Madin–Darby canine kidney (MDCK) cells were obtained from the Institute of Biochemistry and Cell Biology, Shanghai Institute of Biological Science, Chinese Academy of Sciences, China. The LO2 and HEK293T cell lines obtained from the Key Laboratory of Pu-er Tea Science, Ministry of Education, were purchased from Kunming Institute of Zoology, Chinese Academy of Sciences. All cells were cultured in Dulbecco’s modified Eagle’s medium (DMEM; GibcoTM, USA), supplemented with 10% fetal bovine serum (FBS; GibcoTM, USA) and 1% Penicillin-Streptomycin (PS; GibcoTM, USA) at 37°C with 5% CO2 in a humidified atmosphere. The influenza virus A/Puerto Rico/8/34 was obtained from Southern Medical University (Guangzhou, China). The experiments were performed using 100TCID50·ml−1. Ribavirin, a reference antiviral drug, was purchased from Qianjiang Pharmaceutical Co., Ltd., Hubei, China. The 40 tested compounds were dissolved in dimethyl sulfoxide (DMSO; Sigma–Aldrich, USA) at 0.1 M as stock solutions and kept at −80°C. The stock solutions were diluted serially in culture media prior to use. The final concentration of DMSO in testing solutions was always lower than 0.1%. The Trypsin-EDTA solution and 3-(4,5-dimetylthiazol-2-yl)-2,5-diphenyltetrazolium bromide (MTT) were purchased from Biosharp, China.
2.2.2 Initial in Vitro Screening of β-Pinene Derivatives for Antiviral Activities
The MTT method was employed to evaluate the antiviral activities of the β-pinene derivatives against the A/Puerto Rico/8/34 strain of influenza virus. An initial in vitro antiviral screening was conducted at concentrations of 50 and 100 μmol/L to ensure cell viability and enable the compounds to exert their antiviral effects at effective concentrations. These concentrations were selected to minimize potential cytotoxicity while maximizing antiviral efficacy. The compounds were used before and after infection to identify the compounds with antiviral effects. The methodology employed in this study was as follows:
In the first experimental approach, drugs were used before contracting the virus. The MDCK cells in the logarithmic phase were collected and trypsinized with 0.25% trypsin/0.02% EDTA. The cells were then diluted into cell suspensions and inoculated into 96-well tissue culture plates at a concentration of 2 × 105 cells/well. The plates were incubated in CO2 incubators until a confluent monolayer of cells was formed. Next, the cells were treated with the 40 tested compounds at concentrations of 50 and 100 μmol/L. Each compound was tested in triplicate wells. After a 12-h incubation at 37°C, the drug solution was removed. The MDCK cells were then infected with 100TCID50 influenza virus A/PR/8/34 (H1N1) for 1.5 h at 37°C. Following viral inoculation, the viral solution was replaced with a cell maintenance medium, and the cultures were continued at 37°C with 5% CO2. Negative control (no drug) and blank control (no virus, no drug) were included on each plate of cells. When the cells in the negative control group exhibited cytopathic effect (CPE) of up to 80%, 20 µL of 5 mg/mL MTT was added to each well, and the cultures were incubated for 4 h. After incubation, the supernatant was removed and 150 µL of DMSO was added to each well to dissolve the formed formazan crystals. The absorbance (optical density) values of each well were measured using a microplate reader at a wavelength of 570 nm.
For the second experimental approach, the virus was contracted before using drugs. The cells were prepared following the same protocol as described previously. The MDCK cells were infected with a dilution of the H1N1 virus (100TCID50) for 1.5 h at 37°C. After viral incubation, the viral solution was discarded, and the tested compounds at concentrations of 50 and 100 μmol/L were added to the wells. Each compound was tested in triplicate wells. The plates were then transferred to a CO2 incubator and incubated at 37°C. The MTT test was carried out immediately when untreated cells showed CPE of up to 80% as an indicator of viral infection. The MTT test procedure was similar to that described earlier.
The viral inhibition rate was calculated using the following expression:
Inhibition (%) = (average test absorption − average negative control absorption/average blank control absorption − average negative control absorption) × 100%
Compounds that exhibited an inhibition rate of >50% were identified as having some anti-influenza virus activity, and their half-maximal inhibitory concentration (IC50) values (concentration required for 50% inhibition) were determined in the subsequent step.
2.2.3 IC50 Values Calculated for β-Pinene Derivatives with Activity against Influenza Virus
The β-pinene derivatives were serially diluted in concentrations of 3.125, 6.25, 12.5, 25, 50, and 100 µmol/L to determine the IC50 values of the selected compounds. The assay procedure described in Section 2.2.2 was used with ribavirin as a positive control. The IC50 values of the compounds were calculated using the modified Karber method [21].
The cytotoxicity of the compounds on two human cell lines, LO2 and HEK293T, was evaluated using the MTT assay. The LO2 and HEK293T cell lines were cultured and maintained in DMEM. Cells were seeded at a density of 1 × 104 cells/well in a 96-well plate and allowed to attach and grow for 24 h. Upon reaching the desired confluency, 10 μg/mL of the compound was added to the cell culture wells. The cells were then co-incubated with the compound in a CO2 incubator for 24 h. Untreated cell cultures were used as blank controls. After the incubation period, 20 μL of the MTT solution (5 mg/mL) was added to each well and further incubated for 2–4 h. Subsequently, the culture media in the wells were carefully removed, and 50 μL of DMSO per well was added to dissolve the formazan crystals. The absorbance of the wells was measured at 570 nm using a microplate reader. This experiment was repeated three times to ensure reliability. Cell viability was evaluated as the ratio of the absorbance of compound-treated wells to that of untreated control wells. Higher absorbance values indicated greater cell viability, whereas lower values suggested increased cellular toxicity.
Compounds 4a–4l were 3-cyanopyridine derivatives, and they were synthesized efficiently through a multicomponent reaction (MCR) involving aldehydes, ketones, ammonium acetate, and either malononitrile or ethyl cyanoacetate [22]. However, as β-pinene, the starting material, was an alkene, it could not directly participate in the MCR process. Therefore, a prior conversion into (+)-nopinone (compound 2) was required, which possessed the reactivity for the desired reaction. This conversion was accomplished by oxidizing β-pinene with potassium permanganate in acetone, offering a commendable yield of 82% for (+)-nopinone. Then, the reaction of (+)-nopinone with aldehydes 3a–3l, ammonium acetate, and malononitrile in the presence of catalyst ytterbium triflate resulted in 3-cyanopyridines 4a–4l with 52%–87% yields.
Then, myrtanyl acid (6) was synthesized from β-pinene through a two-step process. First, β-pinene underwent a hydroboration oxidation reaction to yield myrtanol (compound 5). Subsequently, myrtanol was oxidized using the Jones reagent to obtain myrtanyl acid. In this study, the hydroboration of β-pinene to generate an organoborane had to be conducted under strictly anhydrous conditions, and oxidation with alkaline hydrogen peroxide yielded myrtanol with 96% yield. However, the myrtanol, with its bridge-ring structure, was prone to ring opening during vigorous oxidation. To overcome this issue and synthesize myrtle acid, we chose to oxidize myrtanol using a Jones reagent in glacial acetic acid of moderate acidity. This method provided a yield of 24.5% for myrtanyl acid.
The synthesis of acylthiourea derivatives (compounds 9a–9k) and amide derivatives (compounds 10a–10o) involved the derivatization of myrtanyl acid based on the substructure splicing principle. A sequence of target compounds was obtained by splicing an acylthiourea moiety or an amide moiety onto the pinane skeleton. First, myrtanyl acid was converted into myrtanyl chloride (compound 7) using oxalyl chloride as the acyl chloride reagent. This intermediate was then treated with potassium thiocyanate to synthesize an isothiocyanate compound (compound 8). Next, the isothiocyanate compound was reacted with a series of amines to give N-aryl-N’-myrtanyl acylthioureas 9a–9g with yields of 81%–83% or N-(4-aryl-thiazolyl-2-yl)-N’-myrtanyl acylthioureas 9h–9k with yields of 8%–11%. In parallel, myrtanyl chloride was reacted directly with various amines to produce a series of N-aryl myrtanyl acid amides 10a–10k with yields of 84%–97% or N-(4-aryl-thiazolyl-2-yl)-myrtanyl acid amides 10l–10o with yields of 16%–28%. Thiourea was reacted with a series of α-bromoacetophenone to introduce thiazole ring into the structures of acylthiourea and amide derivatives. This reaction generated 4-arylthiazol-2-amines, which acted as important intermediates for the previously mentioned reactions.
The molecular structures of the products were confirmed by 1H NMR, 13C NMR, infrared spectroscopy, and MS. The findings were consistent with the expected results, which indicated that the target derivatives 4a–4l, 6, 9a–9k, and 10a–10o were successfully synthesized in this study. The spectral data for these compounds are provided in the Supplementary Materials.
3.2 Antiviral Activity against Influenza Virus A
3.2.1 Preventive Effects of β-Pinene Derivatives on A/PR/8/34 (H1N1) Virus Infection
The anti-influenza A virus activity of β-pinene derivatives was evaluated in vitro using the MTT assay. The results of the blocking effect of pretreatment with β-pinene derivatives on cells infected with influenza virus A/PR/8/34 (H1N1) are shown in Fig. 2.
Figure 2: Preventive effects of β-pinene derivatives on A/PR/8/34 (H1N1) virus infection
As shown in Fig. 2, most β-pinene derivatives did not exhibit a preventive effect against the influenza virus. Only compound 10o showed preventive activity against the H1N1 virus, displaying 49.8% inhibition at a concentration of 50 μmol/L and 55.7% inhibition at a concentration of 100 μmol/L. The data presented in Fig. 2 were analyzed to investigate the relationship between the structures of these derivatives and their preventive activity.
First, in terms of the preventive activity of myrtanyl amide against influenza virus A (H1N1), compound 10e, which substituted the hydrogen at the para-position of the benzene ring with trifluoromethyl, exhibited weak inhibition (17.1%) at a high drug concentration of 100 μmol/L, apart from compound 10o. Examining the structures of compounds 10o and 10e revealed that both contained fluorine atomic substituents. This suggested that the presence of fluorine atomic substituents might contribute to the activity of the derivatives. However, a further observation revealed that compound 10a with two fluorine atom substituents and compound 10d with one fluorine atom substituent exerted an inhibitory effect of −27.2% and −41.7% at a concentration of 100 μmol/L, respectively, demonstrating no preventive activity. This result suggested that the activity of the derivatives might also be related to the number of fluorine atoms. Chernyshov et al. [23] and Jeyaram et al. [24] demonstrated that the introduction of fluorine atoms positively influenced the antiviral activity of the compounds, consistent with our experimental results. Nevertheless, why compound 10o, with only one fluorine atom substitution, displayed significant preventive activity was explored. We hypothesized that this might be attributed to the introduction of the thiazole structure. Consequently, we compared the activity of compound 10l, which incorporated the thiazole structure, with that of compound 10h, which lacked the thiazole structure. The results indicated that the introduction of the thiazole structure moderately improved the preventive activity of the derivatives. This was not surprising because the thiazole structure showed considerable antiviral potential in the last decade, with several studies identifying its efficacy as an antiviral drug [25–27]. Osman et al. [28] also reported the potential of the thiazole structure against influenza virus A (H1N1).
Second, regarding the preventive activity of myrtanyl acylthioureas against virus A (H1N1), only compounds 9c and 9h exhibited weak preventive activity, with inhibitions of 34.8% and 16.1%, respectively, at a concentration of 100 μmol/L. This confirmed our previous assumption that substituting fluorine atoms and introducing thiazole structures improved the preventive activity of the compounds to a certain extent. This was also reflected in 3-cyanopyridine derivatives, as compound 4i, with two fluorine atom substitutions on the benzene ring, displayed the highest inhibition of 34.9% at a concentration of 100 μmol/L among all derivatives in this series.
Third, amide structure, acylthiourea structure, 3-cyanopyridine structure, and carboxylic acid structure exhibited no significant effect on the preventive activity of these derivatives. Moreover, the data did not sufficiently demonstrate the influence of halogen atoms other than fluorine substitutions on the activity. We presumed that the presence of multiple halogen substituents on the benzene ring also facilitated the preventive activity of the derivatives and was not limited to the fluorine atom. The improvement in the preventive activity might be attributed to the introduction of halogen substituents that increased the lipid solubility of the derivatives, leading to the easy absorption of the compounds by the organisms [29].
3.2.2 Therapeutic Effects of β-Pinene Derivatives on A/PR/8/34 (H1N1) Virus Infection
The results of the therapeutic effect of β-pinene derivatives on cells infected with influenza virus A/PR/8/34 (H1N1) in vitro are shown in Fig. 3.
Figure 3: Therapeutic effects of β-pinene derivatives on A/PR/8/34 (H1N1) virus infection
Fig. 3 shows that three β-pinene derivatives 4e, 4i, and 4l exhibited significant antiviral activity with inhibitions of 72.1%, 80.2%, and 58.7%, respectively, at a concentration of 100 μmol/L. Notably, all three derivatives were 3-cyanopyridine derivatives of β-pinene. Similar findings were reported by Wu et al. [30] and Manna et al. [31], indicating that 2-amino-3-cyanopyridine served as a vital bioactive scaffold with antiviral properties along with various other biological activities. Therefore, the 3-cyanopyridine structure holds promise for the development of antiviral drugs. An initial analysis of the structure–activity relationship was conducted to further comprehend the impact of the derivatives’ structure on their antiviral activity.
The therapeutic activity of 3-cyanopyridine derivatives against influenza virus revealed significant variations in the activity of derivatives containing the same substituent at different positions on the benzene ring. This suggested that the position of the substituents within the phenyl group of the 3-cyanopyridine derivative was important in determining its antiviral activity. Moreover, the activity differed when different substituents were introduced at the same position on the benzene ring. However, determining the precise position that leads to higher antiviral activity or which type of substituent is more effective is difficult, implying that the overall structure of the molecule may be necessary for determining antiviral activity. Additionally, the effect of the number of substituents on the activity remains unclear. Although an increase in the number of fluorine atom substituents enhanced the activity, the influence of the substituent position could not be excluded. Notably, compound 4h, with two methoxy substituents, exhibited lower activity compared with compound 4e, which had one methoxy substituent at the C-2 position of the benzene ring.
Myrtanyl acid, (+)-nopinone, and certain myrtanyl acylthioureas and myrtanyl amides were found to exhibit weak inhibitory activity. Interestingly, introducing a trifluoromethyl substituent into the benzene ring in the amide and acylthiourea derivatives resulted in increased potency.
3.2.3 IC50 Values for β-Pinene Derivatives with Activity against Influenza Virus
After preliminary screening 40 β-pinene derivatives, we identified four compounds exhibiting noteworthy anti-influenza A virus activity. The IC50 values were determined using the MTT assay and are presented in Table 1. Notably, among these compounds, only compound 10o demonstrated a preventive effect against cells infected with the influenza A virus, displaying an IC50 value of 47.6 μmol/L. On the contrary, the positive control ribavirin showed no preventive efficacy, consistent with a previous study by Shi et al. [32]. Furthermore, the remaining three compounds exhibited the ability to inhibit virus replication after H1N1 virus infection. Particularly, compound 4e displayed robust anti-influenza A virus activity with an IC50 value of 17.5 μmol/L. Compounds 4i and 4l exhibited similar inhibitory activity, with IC50 values of 32.0 and 34.9 μmol/L, respectively. These promising findings highlighted the potential of introducing a 3-cyanopyridine structure into the pinene skeleton for developing effective antiviral derivatives. These compounds hold promise as potential drug candidates for further exploration in antiviral research, aiming to identify more potent molecules and broaden their applications.
3.2.4 Evaluation of Cytotoxicity
Compound 10o was the sole β-pinene derivative with preventive activity against influenza A virus, whereas compound 4e, among the tested derivatives, exhibited the highest therapeutic activity. However, these compounds could not be classified as effective antiviral drugs without going through cytotoxicity testing. They could be deemed effective antiviral drugs only if they displayed strong antiviral activity and minimal cytotoxicity [33]. Consequently, we assessed the cytotoxicity of compounds 4e and 10o at a concentration of 10 μg/mL against LO2 and HEK293T cells using the MTT assay. The results revealed that neither compound 4e nor compound 10o exhibited cytotoxic effects on LO2 and HEK293T cells at a concentration of 10 μg/mL, as depicted in Fig. 4. This observation suggested that these compounds did not adversely affect cell proliferation or survival at the tested concentration. Normal cell morphology was also observed, indicating healthy and active cells. These findings suggested that compounds 4e and 10o had good safety profiles in LO2 and HEK293T cells.
Figure 4: In vitro cytotoxicity of the two β-pinene derivatives: (a) proportional viability of LO2 cells in response to 10 μg/mL of the compound; (b) image of LO2 cells in response to 10 μg/mL of the control; (c) image of LO2 cells in response to 10 μg/mL of compound 4e; (d) image of LO2 cells in response to 10 μg/mL of compound 10o; (e) proportional viability of HEK293T cells in response to 10 μg/mL of the compound; (f) image of HEK293T cells in response to 10 μg/mL of the control; (g) image of HEK293T cells in response to 10 μg/mL of compound 4e; and (h) image of HEK293T cells in response to 10 μg/mL of compound 10o
The LO2 cells represented a commonly employed human hepatocyte cell line, whereas the HEK293T cells served as a frequently used human embryonic kidney cell line. The liver and kidney, being vital organs within the human body, are relevant in assessing drug metabolism and toxicity. Consequently, choosing these particular cell lines as representative models enabled a more precise evaluation of the influence exerted by pharmaceutical agents on hepatic and renal cellular components, thus ensuring heightened reliability and validity. Furthermore, both the LO2 and HEK293T cell lines have garnered widespread adoption and undergone rigorous validation in the realm of scientific investigation. These cell lines displayed commendable attributes pertaining to their growth dynamics and steadfastness, offering remarkable suitability for experimental cultivation and manipulation strategies, thereby rendering them conducive to facilitating comprehensive analyses.
Although this study tested the cytotoxicity of only two cell lines at a single concentration, these results provided an important basis for the safety of these compounds and also valuable information for their further drug development and toxicity assessment.
This study aimed to evaluate the anti-influenza virus activity of β-pinene derivatives using the MTT method. Among the 40 β-pinene derivatives screened, 4 compounds were identified as having antiviral activity. In addition, subsequent studies can investigate the mechanism of action underlying the antiviral activity exhibited by compounds, demonstrating favorable results. Such an exploration might contribute to our understanding of the mode of action of these compounds. Overall, this study holds significance in both the high-value-added utilization of turpentine and the development of novel anti-influenza virus agents derived from β-pinene derivatives.
Acknowledgement: The authors thank Dr. Jie Yang of Southern Medical University for help conducting in vitro antiviral activity tests. In addition, special thanks to Dr. Lei Wu of Jiangxi Academy of Sciences for help conducting in vitro cytotoxicity tests.
Funding Statement: This work is financially supported by the National Natural Science Foundation of China (Grant Number 32260370), Youth Talent Project of Major Academic and Technical Leaders Training Program of Jiangxi Province (Grant Number 20204BCJL23045), Special Research Project on Camphor Tree (KRPCT) of Jiangxi Forestry Department (Grant Number 2020CXZX07), Innovative Leading Talent Short-Term Project in Natural Science Area of Jiangxi Province (Grant Number jxsq2018102072), the Key Research and Development Program of Jiangxi Province (Grant Number 20192BBFL60014).
Author Contributions: The authors confirm contribution to the paper as follows: study conception and design: Shengliang Liao, Zongde Wang, Zhanqian Song, Shibin Shang; data collection: Yiwen Li, Hongyan Si; analysis and interpretation of results: Yiwen Li, Hongyan Si, Shengliang Liao; draft manuscript preparation: Yiwen Li, Hongyan Si, Peng Wang, Hai Luo, Minggui Shen, Xiaoping Rao. All authors reviewed the results and approved the final version of the manuscript.
Availability of Data and Materials: The authors confirm that the data supporting the findings of this study are available within the article and its supplementary materials.
Conflicts of Interest: The authors declare that they have no conflicts of interest to report regarding the present study.
Supplementary Materials: The supplementary material is available online at https://doi.org/10.32604/jrm.2023.031089.
References
1. Delardas, O., Kechagias, K. S., Pontikos, P. N., Giannos, P. (2022). Socio-economic impacts and challenges of the Coronavirus Pandemic (COVID-19An updated review. Sustainability, 14(15), 9699. [Google Scholar]
2. Uyeki, T. M., Hui, D. S., Zambon, M., Wentworth, D. E., Monto, A. S. (2022). Influenza. Lancet, 400(10353), 693–706. [Google Scholar] [PubMed]
3. Javanian, M., Barary, M., Ghebrehewet, S., Koppolu, V., Vasigala, V. et al. (2021). A brief review of influenza virus infection. Journal of Medical Virology, 93(8), 4638–4646. [Google Scholar] [PubMed]
4. Liu, W. J., Bi, Y., Wang, D., Gao, G. F. (2018). On the centenary of the Spanish flu: Being prepared for the next pandemic. Virologica Sinica, 33(6), 463–466. [Google Scholar] [PubMed]
5. Bhatt, N. A., Singh, A., Samsuddin, S. (2021). A Narrative review of influenza: A seasonal and pandemic disease. European Journal of Molecular & Clinical Medicine, 8(4), 1240. [Google Scholar]
6. Girard, M. P., Tam, J. S., Assossou, O. M., Kieny, M. P. (2010). The 2009 A (H1N1) influenza virus pandemic: A review. Vaccine, 28(31), 4895–4902. [Google Scholar] [PubMed]
7. Ulucay, O., Altunkülah, E., Alkaşi, Ü. (2022). Past to present, epidemiology of SARS-CoV-2 infection and ways of treatment: A review. Kafkas Journal of Medical Sciences, 12(3), 281–291. https://doi.org/10.5505/kjms.2022.90757 [Google Scholar] [CrossRef]
8. Koul, O., Walia, S., Dhaliwal, G. S. (2008). Essential oils as green pesticides: Potential and constraints. Biopesticides International, 4(1), 63–84. [Google Scholar]
9. Cai, J., Yan, R., Shi, J., Chen, J., Long, M. et al. (2022). Antifungal and mycotoxin detoxification ability of essential oils: A review. Phytotherapy Research, 36(1), 62–72. [Google Scholar] [PubMed]
10. Wilkin, P. J., Al-Yozbaki, M., George, A., Gupta, G. K., Wilson, C. M. (2020). The undiscovered potential of essential oils for treating SARS-CoV-2 (COVID-19). Current Pharmaceutical Design, 26(41), 5261–5277. [Google Scholar] [PubMed]
11. Wani, A. R., Yadav, K., Khursheed, A., Rather, M. A. (2021). An updated and comprehensive review of the antiviral potential of essential oils and their chemical constituents with special focus on their mechanism of action against various influenza and coronaviruses. Microbial Pathogenesis, 152, 104620. [Google Scholar] [PubMed]
12. Chen, M., Duan, W. G., Lin, G. S., Fan, Z. T., Wang, X. (2021). Synthesis, antifungal activity, and 3D-QSAR study of novel nopol-derived 1,3,4-thiadiazole-thiourea compounds. Molecules, 26(6), 1708. [Google Scholar] [PubMed]
13. Gao, Y. Q., Hao, J., Li, J., Song, Z. Q., Shang, S. B. (2019). Structural modification of turpentine with natural chiral preservation and low-risk application prospects in crop protection. ACS Omega, 4(4), 6392–6398. [Google Scholar]
14. Zhang, H. M., Wang, J., Chen, Y. X., Xu, S. C., Duan, W. G. et al. (2022). Turpentine-derived sec-p-menthane-1-amine derivatives: Synthesis, herbicidal activity, and 3D-QSAR study. ChemistrySelect, 7(16), e202200618. [Google Scholar]
15. Li, J., Li, J. J., Gao, Y. Q., Shang, S. B., Song, Z. Q. et al. (2016). Taking advantage of a sustainable forest resource in agriculture: A value-added application of volatile turpentine analogues as botanical pesticides based on amphipathic modification and QSAR study. ACS Sustainable Chemistry & Engineering, 4(9), 4685–4691. [Google Scholar]
16. Liao, S. L., Liu, Y., Si, H. Y., Xiao, Z. Q., Fan, G. R. et al. (2017). Hydronopylformamides: Modification of the naturally occurring compound (-)-β-pinene to produce insect repellent candidates against Blattella germanica. Molecules, 22(6), 1004. [Google Scholar] [PubMed]
17. Nikitina, L. E., Kiselev, S. V., Startseva, V. A., Lodochnikova, O. A., Rakhmatullina, A. A. et al. (2019). New aspects of using biologically active thioterpenoids of pinane series. Russian Chemical Bulletin, 68(5), 1031–1035. [Google Scholar]
18. Wang, Y. Y., Wu, C. L., Zhang, Q. J., Shan, Y., Gu, W. et al. (2019). Design, synthesis and biological evaluation of novel β-pinene-based thiazole derivatives as potential anticancer agents via mitochondrial-mediated apoptosis pathway. Bioorganic Chemistry, 84, 468–477. [Google Scholar] [PubMed]
19. Liao, S. L., Shang, S. B., Shen, M. G., Rao, X. P., Si, H. Y. et al. (2016). One-pot synthesis and antimicrobial evaluation of novel 3-cyanopyridine derivatives of (-)-β-pinene. Bioorganic & Medicinal Chemistry Letters, 26(6), 1512–1515. [Google Scholar]
20. Shi, Y. F., Si, H. Y., Wang, P., Chen, S. X., Shang, S. B. et al. (2019). Derivatization of natural compound β-pinene enhances its in vitro antifungal activity against plant pathogens. Molecules, 24(17), 3144. [Google Scholar] [PubMed]
21. Hamilton, M. A., Russo, R. C., Thurston, R. V. (1977). Trimmed Spearman-Karber method for estimating median lethal concentrations in toxicity bioassays. Environmental Science & Technology, 11(7), 714–719. [Google Scholar]
22. Fairoosa, J., Neetha, M., Anilkumar, G. (2021). Recent developments and perspectives in the copper-catalyzed multicomponent synthesis of heterocycles. RSC Advances, 11(6), 3452–3469. [Google Scholar] [PubMed]
23. Chernyshov, V. V., Yarovaya, O. I., Esaulkova, I. L., Sinegubova, E., Borisevich, S. S. et al. (2022). Novel O-acylated amidoximes and substituted 1,2,4-oxadiazoles synthesised from (+)-ketopinic acid possessing potent virus-inhibiting activity against phylogenetically distinct influenza A viruses. Bioorganic & Medicinal Chemistry Letters, 55(12), 128465. [Google Scholar]
24. Jeyaram, R. A., Priyadarzini, T. R. K., Radha, C. A., Shanmugam, N. R. S., Ramakrishnan, C. et al. (2019). Molecular dynamics simulation studies on influenza A virus H5N1 complexed with sialic acid and fluorinated sialic acid. Journal of Biomolecular Structure and Dynamics, 37(18), 4813–4824. [Google Scholar] [PubMed]
25. Sokolova, A. S., Yarovaya, O. I., Bormotov, N. I., Shishkina, L. N., Salakhutdinov, N. F. (2018). Synthesis and antiviral activity of camphor-based 1,3-thiazolidin-4-one and thiazole derivatives as orthopoxvirus-reproduction inhibitors. MedChemComm, 9(10), 1746–1753. [Google Scholar] [PubMed]
26. Abu-Melha, S., Edrees, M. M., Riyadh, S. M., Abdelaziz, M. R., Elfiky, A. A. et al. (2020). Clean grinding technique: A facile synthesis and in silico antiviral activity of hydrazones, pyrazoles, and pyrazines bearing thiazole moiety against SARS-CoV-2 main protease (Mpro). Molecules, 25(19), 4565. [Google Scholar] [PubMed]
27. Ke, S., Li, N., Ke, T., Shi, L., Zhang, Z. et al. (2018). Synthesis and evaluation of steroidal thiazoline conjugates as potential antiviral agents. Future Medicinal Chemistry, 10(22), 2589–2605. [Google Scholar] [PubMed]
28. Osman, H., Yusufzai, S. K., Khan, M. S., Abd Razik, B. M., Sulaiman, O. et al. (2018). New thiazolyl-coumarin hybrids: Design, synthesis, characterization, X-ray crystal structure, antibacterial and antiviral evaluation. Journal of Molecular Structure, 1166, 147–154. [Google Scholar]
29. Jeschke, P. (2010). The unique role of halogen substituents in the design of modern agrochemicals. Pest Management Science: Formerly Pesticide Science, 66(1), 10–27. [Google Scholar]
30. Wu, Y., Wu, T., Huang, Y. (2023). A review: Biological activities of novel cyanopyridine derivatives. Archiv der Pharmazie, e2300067. https://doi.org/10.1002/ardp.202300067 [Google Scholar] [PubMed] [CrossRef]
31. Manna, K., Ghosh, P. S., Das, M., Banik, U., Das, A. (2014). 2-Amino-3-cyanopyridine: A bioactive scaffold. International Journal of Pharmaceutical Sciences and Research, 5(6), 2158–2163. [Google Scholar]
32. Shi, L., Xiong, H., He, J., Deng, H., Li, Q. et al. (2007). Antiviral activity of arbidol against influenza A virus, respiratory syncytial virus, rhinovirus, coxsackie virus and adenovirus in vitro and in vivo. Archives of Virology, 152(8), 1447–1455. [Google Scholar] [PubMed]
33. Pizzi, A. (2021). Tannins medical/pharmacological and related applications: A critical review. Sustainable Chemistry and Pharmacy, 22(12), 100481. [Google Scholar]
Cite This Article
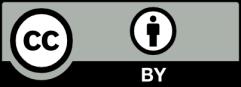